Abstract
Impressive progress has been made since the turn of the century in the field of stem cells. Different types of stem cells have now been isolated from different types of tissues. Pluripotent stem cells are the most promising cell source for organ regeneration. One such cell type is the germline cell-derived pluripotent cell, which is derived from adult spermatogonial stem cells. The germline cell-derived pluripotent stem cells have been obtained from both human and mouse and, importantly, are adult stem cells with embryonic stem cell-like properties that do not require specific manipulations for pluripotency acquisition, hence bypassing problems related to induced pluripotent stem cells and embryonic stem cells. The germline cell-derived pluripotent stem cells have been induced to differentiate into cells deriving from the three germ layers and shown to be functional in vitro. This review will discuss the plasticity of the germline cell-derived pluripotent stem cells and their potential applications in human organ regeneration, with special emphasis on liver regeneration. Potential problems related to their use are also highlighted.
Introduction
Impressive progress has been made since the turn of the century in the field of stem cells and their potential for human regenerative medicine. Stem cells from varying sources are now moving into clinics. Due to the heterogeneity of diseases, as well as to the pathophysiology and anatomy of the targeted organs, there is still no stem cell that fits all requirements.
Embryonic stem (ES) cells, the gold standard for pluripotent stem cells, are derived from the inner cell mass of blastocysts. These cells show the most prominent amenability to in vitro expansion and have been depicted as a highly versatile donor source for basic research and experimental tissue repair.Citation1 They have been shown to participate in chimera formation when microinjected into blastocysts and have been induced to differentiate into multiple cell types. However, ES cell-based therapy has been hampered by moral, legal and ethical dilemma surrounding the use of human embryos for derivation of ES. In addition, the nonautologous origin and tumorigenic potential of ES cells have prompted the finding of alternate sources of pluripotent stem cells that may have applications in human regenerative medicine.
Pluripotency has been conferred to adult cells by somatic cell nuclear transfer, parthenogenesis of unfertilized eggs, reprogramming by cell fusion and by forced expression of key transcription factors or use of small molecules.Citation2–Citation5 The team led by Yamanaka in 2006 first identified four transcription factors (Klf4, Sox2, Oct4 and c-Myc) that could reprogram mouse fibroblasts through retroviral transduction into pluripotent cells.Citation6 This first generation of induced pluripotent stem (iPS) cells presented some concerns, as far as therapeutic value is concerned, due to the use of viral vectors as well as the potent oncogene c-Myc. A series of improvements thereafter followe,d and virus-free iPS cells can now be generated from human adult somatic cells, which brings the use of iPS cells a step closer to the clinic.Citation7–Citation9
Despite the potential of these iPS cells and their similarities to ES cells, significant obstacles to the clinical use of the former exist. The need to introduce transcription factors to induce pluripotency is an ongoing concern, especially if these iPS cells will have to be differentiated in vitro and undergo further modification, for instance, to correct genetic diseases. The technical hurdles concerning generation of iPS cells will surely be resolved in the near future, and the limitations associated with ES are not easily circumvented, hence the run for generating iPS cells from different cell types and with improved methods.Citation10 The accelerated pace of iPS cell research has somewhat overshadowed the potentialities of other adult pluripotent stem cells, which do not require the above-mentioned manipulations to be reprogrammed.
One such cell type is the germline cell-derived pluripotent cell or GPSC (alias maGSC, mGS), which is derived from spermatogonial stem cells (SSCs). This review will discuss about the plasticity of the GPSCs and their potential applications in human organ regeneration, with special emphasis on liver regeneration. Potential problems related to their use are also highlighted.
The Spermatogonial Stem Cells
Spermatogenesis is a complex process involving a wide repertoire of cell types of the testis. It begins 5–7 days and 10–13 years after birth in rodents and humans, respectively, and continues throughout life, hence requiring the continuous presence of a small pool of SSCs. Testicular SSCs attached to the basement membrane of the seminiferous tubules are unipotent and continuously generate differentiating daughter cells for subsequent production of haploid spermatozoa through meiosis.Citation11 Surrounding Sertoli cells provide the necessary architectural support as well as the essential factors for the maintenance of this rare population of stem cells (usually 1 in 3,000 testicular cells). SSCs, despite the absence of specific markers, have been isolated from mouse testis and successfully cultured in vitro for several months. The only way of identifying an SSC thus far is by cell transplant into the seminiferous tubules of infertile recipient mice, as first described by Brinster et al.Citation12,Citation13 Human SSCs have also been isolated and long-term cultures established.Citation14,Citation15 The initial attempts to culture human SSCs in vitro for more than a few passages were unsuccessful probably due to contaminating somatic cells.Citation16 Enrichment of human SSCs using cell surface markers like CD9, GPR125 or integrin α6 allowed cultures of these cells for extended period of time (over six months).Citation14,Citation15,Citation17 Cryopreserving human SSCs, especially before the start of any cancer therapy, is becoming a necessity. Recently, Sadri-Ardekani et al. demonstrated that testicular cells from six males undergoing orchiectomy for prostrate cancer treatment contained SSCs.Citation18 These SSCs were isolated and cultured for up to 15 weeks. The ability to provide long-term culture and propagation of SSCs from humans in vitro is a remarkable leap towards clinical applications.Citation19 SSC culture may also help in understanding human spermatogonial renewal mechanisms.
Once removed from their niche and cultured in vitro, SSCs acquire a larger developmental potential than in vivo. It appears that SSCs can directly transdifferentiate into other cell types when combined with the appropriate mesenchyme.Citation19 SSCs have been injected directly into the renal parenchyma of mature female mice, and fluorescence in situ hybridization technology was used to analyze which types of cells differentiated from SSCs. Interestingly, the Y chrosomome was evidenced in the nucleolus of epithelial cells of the renal tubules as well as podocytes of the glomerulus showing direct differentiation of SSCs into renal cells in vivo.Citation20 However, cell fusion was not verified by these authors, and no in vitro demonstration of differentiation towards this specific lineage exists.
Neonatal mouse SSCs were also shown to directly differentiate into prostatic, uterine and skin epithelium, accompanied by the expression of respective epithelial proteins when combined with the appropriate fetal or neonatal mesenchyme and grafted in vivo.Citation21 It has also been demonstrated that adult testicular cells, isolated from seminiferous tubules, mixed with limiting dilutions of dispersed mammary epithelial cells and injected into epithelium-divested mammary fat pads could adopt mammary epithelial progenitor cell properties, including self-renewal and the production of cell progeny, which differentiate into functional mammary epithelial cells.Citation22 This potential plasticity of SSCs needs to be investigated in more depth.
Germline Cell-Derived Pluripotent Stem Cells
Since the pioneer work by Kanatsu-Shinohara et al., who demonstrated that ES-like cells could be derived from SSCs in long-term in vitro cultures, ardent research has been ongoing in several laboratories to render the use of these cells a valid clinical option.Citation23 Cultured SSCs derived from neonatal testis undergo a spontaneous conversion at low efficiency after 4–7 weeks into ES-like pluripotent stem cells; hence the term germline cell-derived pluripotent stem cells or GPSCs.Citation23–Citation25 The phenomenon of conversion of germline cells to pluripotent ES-like stem cells upon culture stimulation was first observed for cells isolated from the mouse embryo (primordial germ cells) and neonatal testis but has now been successfully reproduced using SSCs from the adult.Citation24–Citation26 GPSCs, which share features of both ES cells and adult stem cells, are of prime importance in tissue regeneration. GPSCs express pluripotent markers, such as Oct3/4, Sox2 and Nanog, and are phenotypically similar to ES cells. Moreover, they produce teratomas when inoculated into mice and form germline chimeras when microinjected into mice blastocysts. GPSCs and ES cells also have similar miRNA profiles.Citation27 However, GPSCs differ from ES cells in their genomic imprinting pattern and in limited gene expression profile due to the differing origin of these two cell types.Citation28,Citation29
Isolation and culture of SSCs and derivation of GPSCs have now been successfully reproduced in humans (termed herein hGPSCs to distinguish these from mouse GPSCs).Citation14 These hGPSCs are similar to human ES cells at cellular and molecular levels and have been continuously passaged for up to 16 months, over more than 40 passages, without decline in colony-forming ability. They could be cryopreserved and thawed with no loss in proliferation or differentiation capacity.Citation17,Citation30,Citation31 However, there is discrepancy between the reports of Conrad et al. and Kossack et al., mainly due to the SSC isolation methods employed.Citation17,Citation30 The former used a three-step procedure to isolate integrin α6+ SSCs from human testicular parenchymas and derived pluripotent ES-like cells (22 out of 22 patients) while the latter cultured testicular cells from testis biopsies using human ES media with subsequent transfer onto mouse embryonic fibroblasts and derived ES-like cells (1 patient) that differed from human ES cells in gene expression, methylation and ability to form teratomas and which were thus considered multipotent. Other groups independently corroborated the above-mentioned reports describing culture of human SSCs and derivation of hGPSCs. Golestaneh et al. obtained pluripotent hGPSCs, which formed the three germ layers upon EBs formation and formed teratoma when injected in nude mice.Citation32 Mizrak et al. obtained multipotent hGPSCs, which did not form extensive teratomas upon injection into immuno-compromized mice.Citation31 Improved and standardized SSC isolation and culture methods need to be established to clarify this issue of pluripotency/multipotency of hGPSCs. Moreover, the identity of the human germ cells, collectively termed spermatogonia or SSCs, that convert to ES-like cells should be better characterized. Importantly, as opposed to iPS cells, the starting cell type for GPSCs is already poised to adopt a pluripotent stem cell fate when stimulated in specific culture conditions. This is indicated by the spontaneous generation of GPSCs from SSCs, without genetic manipulation of the cells. Idealistically, hGPSCs may be generated from SSC cultures and stored in a cell bank after characterization (histocompatibility, donor information, etc.), for differentiation in specific cell types for transplantation. One of the drawbacks is the low efficiency of formation of ES-like cells from SSC cultures in current in vitro conditions. Research on functional small molecules facilitating GPSC generation may help in achieving a solution.Citation33,Citation34 Thus, SSCs, and especially hGPSCs, could become very important tools for human cell-based gene therapy and solicit considerable interest in investigating their differentiative capacity. The attempts at differentiating GPSCs into specific cell types and their potentiality for in vivo use are summarized hereafter.
GPSCs and Liver Regeneration
Liver diseases, in particular chronic hepatitis, commonly caused by hepatitis C (HCV) and B viruses, alcoholism, nonalcoholic fatty liver disease, autoimmune and drug-induced hepatic disorders, affect hundreds of millions of patients worldwide. Since the potential progression from chronic hepatitis to cirrhosis, liver failure and hepatocellular carcinoma is well known, the efficacy of preventing and treating these causative agents is being studied.Citation34
With advances in both surgical technique and immunosuppression regimen, orthotopic liver transplantation (OLT) has become the standard of care for the treatment of patients with end-stage liver disease, and, as a result, the demand for OLT now far exceeds the current donor organ supply. The ongoing organ shortage has reached a critical magnitude, highlighting the burden of recurrent incurable disease. For example, although OLT is not a cure for HCV infection, HCV-related cirrhosis has become the most frequent indication for OLT, with around 50% of liver transplant recipients carrying this diagnosis (43% between 1990–2002 in Turin, Italy).Citation35 Importantly, hepatocytes retain their replicative capacity, unlike other cell types. The liver tissue mass can be restored after two-thirds hepatectomy, as shown by Higgins and Anderson in the 1930s in rat models.Citation36 The human liver also contains a population of stem cells as well as bipotential oval cells that can differentiate into hepatocytes when necessary.Citation37,Citation38 Unfortunately, in severe liver diseases, the hepatocytes' and the liver stem cells' regenerative capacity may be impaired. Thus, much hope of curing the diseased liver has turned towards cell therapy.
The latter has numerous potential advantages when compared to OLT, since transplantable cells can be expanded in vitro and cryopreserved, thus abolishing the limit of organ shortage; can be genetically manipulated to correct inborn errors of metabolism; cryopreserved for future use; infused without major surgery and obtained from the same patient, hence avoiding risk of rejection and need for lifelong immunosuppression.Citation39
Current approaches to cell therapy in humans have involved the use of an extracorporeal liver support system based on primary human hepatocytes and albumin dialysis.Citation40 In other studies, cell transplants in humans have been attempted by the use of human hepatocytes isolated from cadaveric livers.Citation41 However, donor organs are scarce, and the need for lifelong immunosuppressive therapy, as well as the heterogeneity of patients, lack of controls and follow-up duration, greatly limit this approach.
Hepatocyte-like cells have been derived by directed differentiation from bone marrow, adipocytes and mesenchymal stem cells.Citation42 However, when these cells are cryopreserved, a loss in viability is observed.Citation43,Citation44 ES cells have hitherto been the most promising cell source for hepatocyte generation. Both mouse and human ES cells have been used for this purpose.Citation45–Citation47 However, ES cells are allogenic in nature, and transplantation of hepatocyte-like cells generated from them will require immunosuppression, in addition to the other issues described above. Thus, it has become imperative to find alternative sources of cells for liver repair.
Several groups have reported the expression of the early hepatic marker, a fetoprotein, in embryoid bodies (EBs) generated from mouse GPSCs. The directed differentiation of testis-derived pluripotent stem cells into hepatocyte-like cells was also reported, but the characterization of the latter at functional level was not performed.Citation48 We showed, for the first time, that metabolically active hepatocytes, capable of albumin and haptoglobin secretion, urea synthesis, glycogen storage and indocyanine green uptake can be derived from GPSCs in vitro.Citation29 In this study, a large-scale microarray analysis was conducted to compare GPSCs to ES cells during hepatocyte differentiation, and a marked similarity in the gene expression profile between these two cell lines was evidenced. The GPSC-derived hepatocytes were found to closer to fetal hepatocytes (embryonic day 16) than adult hepatocytes (post natal day 1). The in vivo colonization capacity of these cells in mouse models of liver diseases remains to be investigated.
GPSCs are thus a promising tool for the treatment of liver diseases whilst avoiding ethical concerns over embryo use for the production of human pluripotent stem cells. In addition, GPSC-derived hepatocytes may provide the necessary foundation for cell-based therapy for metabolic diseases currently treated by OLT, especially those that can be corrected by only a small amount of functional protein. Hepatocytes derived from GPSCs may prove to be a renewable source of transplantable organs, overcoming the current organ shortage. These cells may also serve as “bridging therapy” between disease diagnosis and organ transplant, as in the case of newborns with genetic defects who have to reach the right age for surgical operation. Moreover, liver transplant mainly requires blood group compatibility, thus bypassing the problem of “perfect” match between donors and recipients.Citation49 In this case, both males and females may benefit from the GPSC-derived hepatocytes. Other advantages of using GPSCs are that these cells can be passaged more than 30 times without changes in karyotype, and that they can be frozen and thawed, much like ES cells. Furthermore, generating and establishing these cell lines as well as making them differentiate into hepatocytes currently takes nearly 6 months (approximately 5 months for culture of SSCs and derivation of GPSCs and 1 month for differentiation of GPSCs into hepatocytes), which, though seemingly a long time, assuming translation to the clinical setting, is far less than the time the patient has to wait when on the transplant list.
GPSCs and Heart Regeneration
Cardiovascular disease remains the leading cause of morbidity and mortality in the western world.Citation50 Heart transplantation is one the most effective treatment for cardiac failure or other cardiomyopathies. The solution for organ scarcity may be offered by cell therapy. Increasing evidence indicates that the mammalian heart contains a population of tissue-specific progenitor cells capable of differentiating into cardiomyocytes, smooth muscle cells and endothelial cells upon request. The heart can also be repopulated by cells from extracardiac sources. Several cell types are being studied as potential sources of cardiomyocytes for cell transplantation therapy and tissue repair in various cardiomyopathy models. Transplantable cell sources identified to date include cardiac progenitor cells,Citation51 fetal cardiomyocytes, mesenchymal stem cells,Citation52 bone marrow cells, ES cells,Citation53 iPS cellsCitation54 and very small embryonic-like cells (VSELS).Citation55 So far, clinical trials on cardiac regeneration involving stem cells have been moderately successful, and thus, it remains unclear which candidate is best for cell transplantation therapy. The inadequacy of these cells to restore the cardiac function of a failing heart has incited the search for alternative therapeutic approaches for substituting lost cardiomyocytes with new functional ones as an alternative to heart transplant. Pluripotent stem cells like GPSCs could offer a substitute to ES cells in cardiomyocytes generation. An interesting study carried out by Guan et al. reported for the first time that GPSCs can be induced to differentiate into functional cardiomyocytes.Citation56 Through molecular, cellular and physiological assays, they demonstrated that GPSC-derived cardiomyocytes had similar properties to those derived from ES cells. Transplantation of GPSCs into the left ventricular free wall of female mice showed that these cells engrafted and were present in heart sections one month post-injection. These cells were able to proliferate and differentiate in the normal heart without teratoma formation. However, the GPSCs differentiated in loco into vascular endothelial and smooth muscle cells. No spontaneous in vivo differentiation into cardiomyocytes were observed in the normal heart. This was probably due to the fact that the local microenvironment is crucial in determining the fate of engrafted cells. Cytokines or growth factors released during myocardial infarction might have promoted the correct differentiation of GPSCs into cardiomyocytes in vivo. Differentiating GPSCs into cardiomyocytes in vitro prior to intramyocardial injection into mice necessitating cardiac regeneration may also circumvent this problem. Another study, carried out by Baba et al., showed that Flk1+ cells from differentiating GPSCs could give rise to mature cardiomyocytes and endothelial cells as efficiently as ES cells.Citation57 The same group also demonstrated that transplantation of Flk1+ GPSCs directly into the heart of ischemic mice improved cardiac function.Citation58 Although 4 weeks after treatment, the number of cardiomyocytes derived from Flk1+ GPSCs were too small to account for the improvement in cardiac function, angiogenesis around ischemic area was enhanced compared to control group, and senescence was also remarkably diminished in the early phase of ischemia.Citation58 The present findings open new possibilities for basic research on cardiac development as well as cardiac regeneration.
GPSCs and Brain Regeneration
The notion of cell replacement therapy to treat neurodegenerative diseases is not new. There are now over two decades of experimental evidence that fetal cell transplantation can be an effective strategy for cellular repair and functional recovery in animal models of neurodegenerative diseases.Citation59 However, the limited availability of fetal tissues, combined with the variable clinical outcomes observed to date, has prompted the search for alternative sources of donor cells for therapeutic intervention. A wide range of stem cells is currently being investigated for cell therapy of neurodegenerative diseases. ES cells have great propensity to form neural precursors, mature neurons and glia upon induction in vitro. It has been shown that dopaminergic neurons from both mouse and human ES cells are capable of integrating host tissue in rodents with Parkinson disease.Citation60–Citation62 Neural stem cell-like populations have been also generated from adult somatic stem cells, like adult human bone marrow stromal cells or skin fibroblasts.Citation63–Citation65 Other studies reported that bone marrow stem cells transplanted into the mouse forebrain after focal occlusion of the middle cerebral artery, an experimental model of ischemic stroke, largely relocate to the infarct penumbra, undergo a process of in situ proliferation and develop into putative cortical interneurons during the early phase of experimental vascular injury.Citation66 GPSCs also show great promise for the generation of neural cell types. Streckfuss-Bomeke et al. have shown that neural progenitors can be obtained from GPSCs under specific culture conditions.Citation59 These progenitors further matured into functional neurons (GABAergic, glutamatergic, serotonergic and dopaminergic neurons) and glial cells (astrocytes and oligodendrocytes). Electrophysiological recordings of passive and active membrane properties and postsynaptic currents demonstrated the maturation of GPSC-derived precursor cells into functional cells.Citation59 Glaser et al. also showed that GPSC-derived neurons generate active functional networks, which use both GABAergic synaptic transmission and engage in synchronized oscillatory activity.Citation1 Another interesting observation was that GPSC-derived oligodendrocytes undergo full maturation and ensheathe host axons in myelin-deficient tissue in organotypic slice cultures of the myelin-deficient rat cerebellum. GPSC-derived neural cells thus constitute a promising cell source for the treatment of many different nervous system disorders. Although GPSC-derived neurons are functional in vitro, their potential to engraft into the damaged host brain and function as neurons after transplantation remains to be demonstrated. Besides their potential for autologous cell therapy, the neural differentiation of GPSCs opens a new window for basic research on neural development and neural regeneration.
GPSCs and Hematopoietic Potential
Hematopoietic stem cell (HSC) transplantation has been widely used for treating hematological malignancies and inherited disorders. Peripheral blood and cord blood stem cells as well as bone marrow cells have been intensively studied and shown to be effective for clinical use. Recently, ES cells have been proposed as an alternative candidate source of HSCs. However, due to the problems associated with transplantation of ES-derived cells, attempts to derive hematopoietic cells from GPSCs have been made. GPSCs have been shown to differentiate, on OP9 cells, into CD45+ hematopoietic cells, including Gr-1+Mac1+ myeloid cells and Ter119+ erythroid cells.Citation23 In a recent report, Yoshimoto et al. reported the generation of multipotent hematopoietic progenitor cells with myeloid and lymphoid potential emerging from FLK1+-differentiating GPSCs using the OP9 feeder cell system and described the localization of GPSC-derived hematopoietic cells in the bone marrow cavity after intra-bone marrow injection in immunodeficient mice.Citation67 However, the GPSC-derived hematopoietic cells could engraft in the host but did not proliferate or display multilineage repopulating ability in vivo. Thus, much optimization needs to be done in the field of hematopoietic research, but these data serve as a prelude to more promising results in the future.
Potentialities of Human GPSCs
Differentiation studies on hGPSCs are still in their early days, but it has been demonstrated that hGPSCs are capable of differentiating in vitro into derivatives of all three germ layers. Conrad et al. examined teratoma formation efficiency of hGPSCs derived from eight patients by injecting cells intramuscularly or subcutaneously in the neck region of adult nude mice.Citation17 Teratomas that formed after 6–8 weeks contained foci with derivatives of endodermal, mesodermal and ectodermal germ layers, which was confirmed by detailed analysis of tissue sections. These authors also showed that hGPSCs can be differentiated morphologically in vitro into myogenic, osteogenic, pancreatic and neural lineages. Moreover, the hGPSC-derived pancreatic cells also produced insulin-, glucagon- and c-peptide-positive cells. The independent hGPSC cell line generated by Kossack et al. also gave rise spontaneously to the three germ layers upon EBs formation.Citation30 However, no extensive teratoma formation was found when these cells were injected under the kidney capsule of SCID mice. This discrepancy in teratoma formation by hGPSCs between these two studies may be due to the differing cell injection sites and methods used.Citation68 Human GPSCs can also be induced to differentiate into NES+, MAP2+ and TUBIII+ neural linages after 6 weeks' culture in neural cell-specific media.Citation30 Moreover, hGPSCs were also induced to differentiating into pancreatic β cells, which were shown to produce insulin in response to glucose and osteogenic-differentiated cells that showed deposition of phosphate and calcium, demonstrating their functional capacity.Citation31 The hGPSCs are thus extremely promising, and their full potentiality still needs to be investigated, first, at the preclinical level in vivo in mouse models, then in clinical trials on humans.
Conclusions
The discovery of adult GPSCs and their differentiation plasticity offers great promise for human regenerative medicine whilst avoiding ethical concerns over embryo use for production of pluripotent human stem cells. And, most importantly, no viral vectors or special manipulations are required for “reprogramming” these adult stem cells into pluripotent stem cells.
There is good reason to think that GPSCs, due to their ES cell-like plasticity, can be induced to differentiate into cell types other than those described above (summarized in ) under specific culture conditions.
There are, however, several concerns that need to be addressed before a clinical trial with hGPSC-derived cells can be initiated. First, human SSC isolation and hGPSC derivation methods need to be standardized; second, the differentiation capacity of hGPSCs needs to be fully investigated; third, participation of GPSCs in tissue regeneration has to be correctly assessed in vivo in rodents; fourth, being uniparental, GPSCs have silenced H19 imprinted gene on both alleles. H19 is a tumor suppressor gene, and alteration in its expression is involved in the pathogenesis of some human tumors.Citation69 Finally, more progress is needed in terms of allogenic hGPSC transplantation.
Assuming translation of the SSC/GPSC system to humans, the conversion of cultured adult SSCs into cells with pluripotent features holds great therapeutic potential for degenerative diseases. The availability of compatible, pluripotent cells for transplantation from simple biopsies would circumvent immunological problems associated with regular human ES cell-based technology.
However, like ES and iPS cells, these GPSCs are target of lymphokine-activated killer (LAK) cells and natural killer (NK) cells in vitro. Moreover, NK cells were able to kill these pluripotent stem cells in vivo too.Citation70 Interestingly, differentiation induced resistance to NK and LAK cells, indicating the importance of eliminating residual pluripotent stem cells from differentiated ones before cell transplant can be considered.
In conclusion, GPSCs may offer a safer means of generating pluripotent cells than other adult cells and provide new opportunities for getting patient-specific regenerative therapy. Cell therapy with hGPSCs will surely bring great improvements over the current organ regeneration strategies mentioned above. An “ethical” stem cell has probably been found.
Key Messages
As an alternative to ES cell-based therapy, which has been hampered by moral, legal and ethical dilemma, there are the germline cell-derived pluripotent cells or GPSCs, which are ES-like cells derived from spermatogonial stem cells.
GPSCs derived from mice and humans are naturally pluripotent/multipotent, and share features of both ES cells and adult stem cells, hence rendering them very important in tissue regeneration.
Metabolically active hepatocytes, capable of albumin and haptoglobin secretion, urea synthesis, glycogen storage and indocyanine green uptake have been derived from GPSCs in vitro and may be used for cell therapy of the liver.
Other promising applications of GPSCs have been reported for heart and brain regeneration and in hematology.
Abbreviations
EBs | = | embryoid bodies |
ES | = | embryonic stem |
GPSCs | = | germline cell-derived pluripotent stem cells |
hGPSCs | = | human GPSCs |
iPS | = | induced pluripotent stem |
LAK | = | lymphokine-activated killer |
MaGSCs | = | multipotent adult germline stem cells |
mGS | = | multipotent germline stem |
NK | = | natural killer |
OLT | = | orthotopic liver transplantation |
SSC | = | spermatogonial stem cells |
VSELS | = | very small embryonic-like cells |
Figures and Tables
Figure 1 Differentiative potential of GPSCs. SSCs can be cryopreserved for restoring fertility in patients undergoing chemotherapy. When cultured, SSCs give rise to ES-like colonies (GPSCs) that can be induced to differentiate into cells of different lineages. Hepatocytes generated from GPSCs are functional. A PAS-positive hepatocyte is shown.
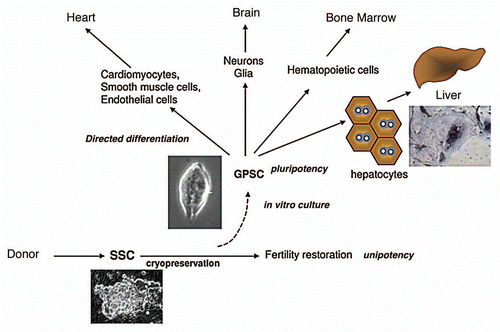
Acknowledgments
Our studies were supported by grants from PI-STEM (Regione Piemonte), PRIN 2008. Special thanks to Letizia De Chiara and Francesca Barbieri for experimental help.
References
- Glaser T, Opitz T, Kischlat T, Konang R, Sasse P, Fleischmann BK, et al. Adult germ line stem cells as a source of functional neurons and glia. Stem Cells 2008; 26:2434 - 2443
- Perry AC. Progress in human somatic-cell nuclear transfer. N Engl J Med 2005; 353:87 - 88
- Lyssiotis CA, Foreman RK, Staerk J, Garcia M, Mathur D, Markoulaki S, et al. Reprogramming of murine fibroblasts to induced pluripotent stem cells with chemical complementation of Klf4. Proc Natl Acad Sci USA 2009; 106:8912 - 8917
- Shi Y, Desponts C, Do JT, Hahm HS, Scholer HR, Ding S. Induction of pluripotent stem cells from mouse embryonic fibroblasts by Oct4 and Klf4 with small-molecule compounds. Cell Stem Cell 2008; 3:568 - 574
- Kim K, Ng K, Rugg-Gunn PJ, Shieh JH, Kirak O, Jaenisch R, et al. Recombination signatures distinguish embryonic stem cells derived by parthenogenesis and somatic cell nuclear transfer. Cell Stem Cell 2007; 1:346 - 352
- Takahashi K, Yamanaka S. Induction of pluripotent stem cells from mouse embryonic and adult fibroblast cultures by defined factors. Cell 2006; 126:663 - 676
- Yu J, Hu K, Smuga-Otto K, Tian S, Stewart R, Slukvin II, et al. Human induced pluripotent stem cells free of vector and transgene sequences. Science 2009; 324:797 - 801
- Kaji K, Norrby K, Paca A, Mileikovsky M, Mohseni P, Woltjen K. Virus-free induction of pluripotency and subsequent excision of reprogramming factors. Nature 2009; 458:771 - 775
- Lengner CJ. iPS cell technology in regenerative medicine. Ann N Y Acad Sci 2010; 1192:38 - 44
- Hanna JH, Saha K, Jaenisch R. Pluripotency and cellular reprogramming: facts, hypotheses, unresolved issues. Cell 2010; 143:508 - 525
- Simon L, Hess RA, Cooke PS. Spermatogonial stem cells, in vivo transdifferentiation and human regenerative medicine. Expert Opin Biol Ther 2010; 10:519 - 530
- Brinster RL, Zimmermann JW. Spermatogenesis following male germ-cell transplantation. Proc Natl Acad Sci USA 1994; 91:11298 - 11302
- Brinster RL, Avarbock MR. Germline transmission of donor haplotype following spermatogonial transplantation. Proc Natl Acad Sci USA 1994; 91:11303 - 11307
- Lim JJ, Sung SY, Kim HJ, Song SH, Hong JY, Yoon TK, et al. Long-term proliferation and characterization of human spermatogonial stem cells obtained from obstructive and non-obstructive azoospermia under exogenous feeder-free culture conditions. Cell Prolif 2010; 43:405 - 417
- He Z, Kokkinaki M, Jiang J, Dobrinski I, Dym M. Isolation, characterization and culture of human spermatogonia. Biol Reprod 2010; 82:363 - 372
- Lee DR, Kim KS, Yang YH, Oh HS, Lee SH, Chung TG, et al. Isolation of male germ stem cell-like cells from testicular tissue of non-obstructive azoospermic patients and differentiation into haploid male germ cells in vitro. Hum Reprod 2006; 21:471 - 476
- Conrad S, Renninger M, Hennenlotter J, Wiesner T, Just L, Bonin M, et al. Generation of pluripotent stem cells from adult human testis. Nature 2008; 456:344 - 349
- Sadri-Ardekani H, Mizrak SC, van Daalen SK, Korver CM, Roepers-Gajadien HL, Koruji M, et al. Propagation of human spermatogonial stem cells in vitro. JAMA 2009; 302:2127 - 2134
- Hwang K, Lamb DJ. New advances on the expansion and storage of human spermatogonial stem cells. Curr Opin Urol 2010; 20:510 - 514
- Wu DP, He DL, Li X, Liu ZH. Differentiations of transplanted mouse spermatogonial stem cells in the adult mouse renal parenchyma in vivo. Acta Pharmacol Sin 2008; 29:1029 - 1034
- Simon L, Ekman GC, Kostereva N, Zhang Z, Hess RA, Hofmann MC, et al. Direct transdifferentiation of stem/progenitor spermatogonia into reproductive and nonreproductive tissues of all germ layers. Stem Cells 2009; 27:1666 - 1675
- Boulanger CA, Mack DL, Booth BW, Smith GH. Interaction with the mammary microenvironment redirects spermatogenic cell fate in vivo. Proc Natl Acad Sci USA 2007; 104:3871 - 3876
- Kanatsu-Shinohara M, Inoue K, Lee J, Yoshimoto M, Ogonuki N, Miki H, et al. Generation of pluripotent stem cells from neonatal mouse testis. Cell 2004; 119:1001 - 1012
- Guan K, Nayernia K, Maier LS, Wagner S, Dressel R, Lee JH, et al. Pluripotency of spermatogonial stem cells from adult mouse testis. Nature 2006; 440:1199 - 1203
- Kanatsu-Shinohara M, Lee J, Inoue K, Ogonuki N, Miki H, Toyokuni S, et al. Pluripotency of a single spermatogonial stem cell in mice. Biol Reprod 2008; 78:681 - 687
- Seandel M, James D, Shmelkov SV, Falciatori I, Kim J, Chavala S, et al. Generation of functional multipotent adult stem cells from GPR125+ germline progenitors. Nature 2007; 449:346 - 350
- Zovoilis A, Nolte J, Drusenheimer N, Zechner U, Hada H, Guan K, et al. Multipotent adult germline stem cells and embryonic stem cells have similar microRNA profiles. Mol Hum Reprod 2008; 14:521 - 529
- Ko K, Tapia N, Wu G, Kim JB, Bravo MJ, Sasse P, et al. Induction of pluripotency in adult unipotent germline stem cells. Cell Stem Cell 2009; 5:87 - 96
- Fagoonee S, Hobbs RM, De Chiara L, Cantarella D, Piro RM, Tolosano E, et al. Generation of functional hepatocytes from mouse germ line cell-derived pluripotent stem cells in vitro. Stem Cells Dev 2010; 19:1183 - 1194
- Kossack N, Meneses J, Shefi S, Nguyen HN, Chavez S, Nicholas C, et al. Isolation and characterization of pluripotent human spermatogonial stem cell-derived cells. Stem Cells 2009; 27:138 - 149
- Mizrak SC, Chikhovskaya JV, Sadri-Ardekani H, van Daalen S, Korver CM, Hovingh SE, et al. Embryonic stem cell-like cells derived from adult human testis. Hum Reprod 2010; 25:158 - 167
- Golestaneh N, Kokkinaki M, Pant D, Jiang J, DeStefano D, Fernandez-Bueno C, et al. Pluripotent stem cells derived from adult human testes. Stem Cells Dev 2009; 18:1115 - 1126
- Yuan X, Wan H, Zhao X, Zhu S, Zhou Q, Ding S. Combined Chemical Treatment Enables Oct4-Induced Reprogramming from Mouse Embryonic Fibroblasts. Stem Cells 2011;
- Chen Z, Qi LZ, Zeng R, Li HY, Dai LJ. Stem cells and hepatic cirrhosis. Panminerva Med 2010; 52:149 - 165
- Salizzoni M, Cerutti E, Romagnoli R, Lupo F, Franchello A, Zamboni F, et al. The first one thousand liver transplants in Turin: a single-center experience in Italy. Transpl Int 2005; 18:1328 - 1335
- Brezillon N, Kremsdorf D, Weiss MC. Cell therapy for the diseased liver: from stem cell biology to novel models for hepatotropic human pathogens. Dis Model Mech 2008; 1:113 - 130
- Herrera MB, Bruno S, Buttiglieri S, Tetta C, Gatti S, Deregibus MC, et al. Isolation and characterization of a stem cell population from adult human liver. Stem Cells 2006; 24:2840 - 2850
- Oh SH, Hatch HM, Petersen BE. Hepatic oval ‘stem’ cell in liver regeneration. Semin Cell Dev Biol 2002; 13:405 - 409
- Piscaglia AC, Campanale M, Gasbarrini A, Gasbarrini G. Stem cell-based therapies for liver diseases: state of the art and new perspectives. Stem Cells Int 2010; 25946
- Sauer IM, Zeilinger K, Pless G, Kardassis D, Theruvath T, Pascher A, et al. Extracorporeal liver support based on primary human liver cells and albumin dialysis—treatment of a patient with primary graft non-function. J Hepatol 2003; 39:649 - 653
- Schneider A, Attaran M, Meier PN, Strassburg C, Manns MP, Ott M, et al. Hepatocyte transplantation in an acute liver failure due to mushroom poisoning. Transplantation 2006; 82:1115 - 1116
- Duncan AW, Dorrell C, Grompe M. Stem cells and liver regeneration. Gastroenterology 2009; 137:466 - 481
- Carvalho KA, Cury CC, Oliveira L, Cattaned RI, Malvezzi M, Francisco JC, et al. Evaluation of bone marrow mesenchymal stem cell standard cryopreservation procedure efficiency. Transplant Proc 2008; 40:839 - 841
- Fuller R, DRV. The effect of two different freezing methods on the immediate post-thaw membrane integrity of adipose tissue derived stem cells. Int J Heat Mass Transf 2008; 51:5650 - 5654
- Cho CH, Parashurama N, Park EY, Suganuma K, Nahmias Y, Park J, et al. Homogeneous differentiation of hepatocyte-like cells from embryonic stem cells: applications for the treatment of liver failure. FASEB J 2008; 22:898 - 909
- Agarwal S, Holton KL, Lanza R. Efficient differentiation of functional hepatocytes from human embryonic stem cells. Stem Cells 2008; 26:1117 - 1127
- Hay DC, Zhao D, Ross A, Mandalam R, Lebkowski J, Cui W. Direct differentiation of human embryonic stem cells to hepatocyte-like cells exhibiting functional activities. Cloning Stem Cells 2007; 9:51 - 62
- Loya K, Eggenschwiler R, Ko K, Sgodda M, Andre F, Bleidissel M, et al. Hepatic differentiation of pluripotent stem cells. Biol Chem 2009; 390:1047 - 1055
- Navarro V, Herrine S, Katopes C, Colombe B, Spain CV. The effect of HLA class I (A and B) and class II (DR) compatibility on liver transplantation outcomes: an analysis of the OPTN database. Liver Transpl 2006; 12:652 - 658
- Gonzales C, Pedrazzini T. Progenitor cell therapy for heart disease. Exp Cell Res 2009; 315:3077 - 3085
- Rosenblatt-Velin N, Lepore MG, Cartoni C, Beermann F, Pedrazzini T. FGF-2 controls the differentiation of resident cardiac precursors into functional cardiomyocytes. J Clin Invest 2005; 115:1724 - 1733
- Psaltis PJ, Zannettino AC, Worthley SG, Gronthos S. Concise review: mesenchymal stromal cells: potential for cardiovascular repair. Stem Cells 2008; 26:2201 - 2210
- Kolossov E, Bostani T, Roell W, Breitbach M, Pillekamp F, Nygren JM, et al. Engraftment of engineered ES cell-derived cardiomyocytes but not BM cells restores contractile function to the infarcted myocardium. J Exp Med 2006; 203:2315 - 2327
- Zhang J, Wilson GF, Soerens AG, Koonce CH, Yu J, Palecek SP, et al. Functional cardiomyocytes derived from human induced pluripotent stem cells. Circ Res 2009; 104:30 - 41
- Wojakowski W, Kucia M, Zuba-Surma E, Jadczyk T, Ksiazek B, Ratajczak MZ, et al. Very small embryonic-like stem cells in cardiovascular repair. Pharmacol Ther 2011; 129:21 - 28
- Guan K, Wagner S, Unsold B, Maier LS, Kaiser D, Hemmerlein B, et al. Generation of functional cardiomyocytes from adult mouse spermatogonial stem cells. Circ Res 2007; 100:1615 - 1625
- Baba S, Heike T, Umeda K, Iwasa T, Kaichi S, Hiraumi Y, et al. Generation of cardiac and endothelial cells from neonatal mouse testis-derived multipotent germline stem cells. Stem Cells 2007; 25:1375 - 1383
- Iwasa T, Baba S, Doi H, Kaichi S, Yokoo N, Mima T, et al. Neonatal mouse testis-derived multipotent germline stem cells improve the cardiac function of acute ischemic heart mouse model. Biochem Biophys Res Commun 2010; 400:27 - 33
- Streckfuss-Bomeke K, Vlasov A, Hulsmann S, Yin D, Nayernia K, Engel W, et al. Generation of functional neurons and glia from multipotent adult mouse germline stem cells. Stem Cell Res 2009; 2:139 - 154
- Kim JH, Auerbach JM, Rodriguez-Gomez JA, Velasco I, Gavin D, Lumelsky N, et al. Dopamine neurons derived from embryonic stem cells function in an animal model of Parkinson's disease. Nature 2002; 418:50 - 56
- Roy NS, Cleren C, Singh SK, Yang L, Beal MF, Goldman SA. Functional engraftment of human ES cell-derived dopaminergic neurons enriched by coculture with telomerase-immortalized midbrain astrocytes. Nat Med 2006; 12:1259 - 1268
- Yang D, Zhang ZJ, Oldenburg M, Ayala M, Zhang SC. Human embryonic stem cell-derived dopaminergic neurons reverse functional deficit in parkinsonian rats. Stem Cells 2008; 26:55 - 63
- Woodbury D, Schwarz EJ, Prockop DJ, Black IB. Adult rat and human bone marrow stromal cells differentiate into neurons. J Neurosci Res 2000; 61:364 - 370
- Vierbuchen T, Ostermeier A, Pang ZP, Kokubu Y, Sudhof TC, Wernig M. Direct conversion of fibroblasts to functional neurons by defined factors. Nature 2010; 463:1035 - 1041
- Qi X, Shao M, Peng H, Bi Z, Su Z, Li H. In vitro differentiation of bone marrow stromal cells into neurons and glial cells and differential protein expression in a two-compartment bone marrow stromal cell/neuron co-culture system. J Clin Neurosci 2010; 17:908 - 913
- Zhang XM, Du F, Yang D, Yu CJ, Huang XN, Liu W, et al. Transplanted bone marrow stem cells relocate to infarct penumbra and co-express endogenous proliferative and immature neuronal markers in a mouse model of ischemic cerebral stroke. BMC Neurosci 2010; 11:138
- Yoshimoto M, Heike T, Chang H, Kanatsu-Shinohara M, Baba S, Varnau JT, et al. Bone marrow engraftment but limited expansion of hematopoietic cells from multipotent germline stem cells derived from neonatal mouse testis. Exp Hematol 2009; 37:1400 - 1410
- Hentze H, Soong PL, Wang ST, Phillips BW, Putti TC, Dunn NR. Teratoma formation by human embryonic stem cells: evaluation of essential parameters for future safety studies. Stem Cell Res 2009; 2:198 - 210
- Elkin M, Shevelev A, Schulze E, Tykocinsky M, Cooper M, Ariel I, et al. The expression of the imprinted H19 and IGF-2 genes in human bladder carcinoma. FEBS Lett 1995; 374:57 - 61
- Dressel R, Nolte J, Elsner L, Novota P, Guan K, Streckfuss-Bomeke K, et al. Pluripotent stem cells are highly susceptible targets for syngeneic, allogeneic and xenogeneic natural killer cells. FASEB J 2010; 24:2164 - 2177