Abstract
The yeast prion [PSI+] represents an aggregated state of the translational release factor Sup35 (eRF3) and deprives termination complexes of functional Sup35, resulting in nonsense codon suppression. Protein-remodeling factor Hsp104 is involved in thermotolerance and [PSI+] propagation, however the structure-and-function relationship of Hsp104 for [PSI+] remains unclear. In this study, we engineered 58 chromosomal hsp104 mutants that affect residues considered structurally or functionally relevant to Hsp104 remodeling activity, yet most remain to be examined for their significance to [PSI+] in the same genetic background. Many of these hsp104 mutants were affected both in thermotolerance and [PSI+] propagation. However, nine mutants were impaired exclusively for [PSI+], while two mutants were impaired exclusively for thermotolerance. Mutations exclusively affecting [PSI+] are clustered around the lateral channel of the Hsp104 hexamer. These findings suggest that Hsp104 possesses shared as well as distinct remodeling activities for stress-induced protein aggregates and [PSI+] prion aggregates and that the lateral channel plays a role specific to [PSI+] prion propagation.
Introduction
Protein-remodeling factor Hsp104 is a member of the ClpB family of AAA+ proteins (ATPases associated with various cellular activities; for a review see ref. Citation1), and is a major heat-inducible protein required for thermotolerance in S. cerevisiae.Citation2 Hsp104 disassembles protein aggregates formed from denatured proteins under stress (e.g., heat-shock) conditions and thereby increases survival.Citation3,Citation4 The Hsp104/ClpB family protein forms ring-shaped hexamers with a central pore.Citation5,Citation6 Recent data show that peptides transit the pore of Hsp104 and ClpB during protein disaggregation.Citation7,Citation8 In addition, Hsp104 is also known to be required for propagation of yeast prions [PSI+], [URE3] and [PIN+]/[RNQ+].Citation9–Citation11 Coupled with ATP hydrolysis, Hsp104 functions to break up large [PSI+] (and probably other prion) aggregates for resolubilization, producing seeds that are transmissible to daughter cells during cell division.Citation12 A loss-of-function in Hsp104 eliminates [PSI+], [URE3] and [PIN+].Citation9–Citation11
The yeast prion [PSI+]Citation13,Citation14 is the amyloid like structureCitation15–Citation17 of the polypeptide release factor Sup35 that is essential for terminating protein synthesis at stop codonsCitation18,Citation19 (for a review see Ref. Citation20). When Sup35 is in the [PSI+] aggregated state, ribosomes often fail to release polypeptides at stop codons, causing a non-Mendelian trait easily detected by nonsense suppression.Citation21–Citation23
Although it is evident that the disaggregation activity of Hsp104 is required for [PSI+], it is not conclusive whether this sole activity of Hsp104 is sufficient. In this regard, an alternative view explains that an additional and distinct activity of Hsp104 to nucleate prion formation is required for [PSI+]Citation24 (reviewed in ref. Citation25). In this model, Hsp104 acts on the soluble prion domain and catalyzes assembly of critical oligomeric intermediates that nucleates fibrillization. In addition, it is not conclusive whether stress-induced protein aggregates and prion aggregates are processed by Hsp104 through the same mechanism. Rather, there is an alternative view that different underlying mechanisms act in the recognition of the different aggregates structures.Citation25 In the literature, there are many hsp104 mutations isolated that have been investigated for Hsp104 remodeling activity in vivo and in vitro. However, of those, only a limited number have been examined for their effects on prion propagation. Therefore, in spite of these mutations and the solved tertiary structure of Hsp104-homolog T. thermophilus ClpB,Citation6 a structure-and-function relationship for Hsp104 required for [PSI+] propagation remains poorly understood. Moreover, previous collections of hsp104 mutants are largely manipulated in an extra-chromosomal copy of the HSP104 gene, which does not reflect the natural situation required for [PSI+] propagation. For these reasons, we engineered 58 single amino acid substitutions in hsp104 and recombined them into the chromosomal wild-type allele of HSP104. These isogenic hsp104 mutants were thoroughly investigated for thermotolerance and [PSI+] propagation.
Materials and Methods
Media and transformation.
Yeast were grown on YPD (1% yeast extract, 2% polypeptone and 2% dextrose), or SC (0.67% yeast nitrogen base without amino acid [DIFCO], 2% dextrose) supplemented with adenine, leucine, uracil, histidine and tryptophan, if required. Transformations were preformed using Frozen-EZ Yeast Transformation IITM kit (ZYMO Research) according to the manufacturer's instructions.
Immunoblotting.
The Western blot analysis was performed as described previously using rabbit anti-Hsp104 antibody (Affinity Bioreagents).Citation26
Strains and plasmids.
S. cerevisiae strains used in this study are listed in (). The hsp104 alleles were derived by primer mutagenesis using the primers listed in () using pUC19-HSP104-LEU2 as template. In addition, C209Y, G212D, A220T, G259D, P389L, L462R, P557L, G661D and D704N alleles were cloned from original mutants by genomic PCR.Citation27 The plasmid carrying the hsp104 whole deletion (Δhsp104) was described previously.Citation27 These alleles replaced chromosomal HSP104 as follows. pUC19-HSP104-LEU2 carries HSP104 from nucleotide position -464 (counted from the transcript start site) to 3327 and the LEU2 segment is inserted in the HSP104 terminator region.Citation27 Fragments containing the HSP104 or its derived mutant alleles and LEU2 was cut with BamHI and SphI. These fragments were transformed into strains subject to gene replacement, and Leu+ transformants were selected. Replacement of hsp104 for HSP104 was confirmed by DNA sequencing.
Tetrad analysis.
Heterozygous diploids were obtained by crossing hsp104 derivatives of AT3 to NPK151 (wild-type HSP104 [PSI+]) or NPK175 (wild-type HSP104 [psi-]) strains. The resulting diploids were cultured on YPD+ade for 1 day and sporulated on 2% potassium acetate for 3 to 4 days. Spores were suspended in buffer (1 M Sorbitol, 50 mM potassium phosphate, pH 7.5, 14 mM β-mercaptoethanol) supplemented with 6 µg/ml lyticase and incubated at 30°C for 40 minutes. The resulting asci were subjected to tetrad manipulation.
Thermotolerance.
Experiments were done as previously described.Citation28 Briefly cells were grown to mid-log phase (OD600 = 0.8–1.0) in YPD+ade at 30°C, and were then incubated at 37°C for 60 minutes to induce Hsp104. After this Hsp104 induction, cultures were transferred to 50°C for 20 minutes. Aliquots of cells were transferred to ice immediately after and the percentage of surviving cells, relative to a culture without heat shock induction, was determined by titration on YPD+ade media.
Results
Construction of a comprehensive repertoire of chromosomal hsp104 mutants.
To investigate the structure-and-function relationship of Hsp104 for [PSI+] propagation, we first generated 58 single mutations in the hsp104 gene, cloned on plasmid pUC19-HSP104-LEU2, and then used them to replace the wild-type HSP104 allele in the S. cerevisiae chromosome by gene replacement (see Materials and Methods). In brief, these 58 mutations caused single amino acid changes at residues that have either been experimentally validated or are thought to be putatively important for Hsp104/ClpB activity in thermotolerance,Citation7,Citation27–Citation34 refolding in vitro,Citation3,Citation28,Citation31,Citation35–Citation37 or structural organization and hexameric assemblyCitation7,Citation28,Citation31–Citation33,Citation35,Citation37,Citation38 (). Many of these mutations, however, were not reported to affect [PSI+] propagation.
Plasmid pUC19-HSP104-LEU2 contains wild-type HSP104 sequence (including its 5′- and 3′-flanking sequences), and the LEU2 selection marker, inserted into the 3'-flanking sequence (the resulting construct is referred to as HSP104<<LEU2). Designed hsp104 mutations were introduced into pUC19-HSP104-LEU2 by PCR using the indicated primers (see ). BamHI-SphI fragments of pUC19-HSP104-LEU2 and its HSP104 mutant derivatives were transformed into the [PSI+] indicator strain AT3 (ade1-14 leu2Δ). Leu+ transformants were selected and those in which the wild-type chromosome HSP104 was replaced by HSP104<<LEU2 and hsp104<<LEU2 alleles by double homologous recombination at sites upstream of hsp104 mutations and downstream of LEU2 were confirmed by sequencing DNA fragments amplified by PCR (data not shown).
Effects of hsp104 mutations on [PSI+] propagation.
When Sup35 is in the [PSI+] state, ribosomes often fail to release polypeptides at stop codons, causing a non-Mendelian trait easily detected by nonsense suppression.Citation21–Citation23 Auxotrophic markers such as ade1-14 (nonsense allele) have been widely used to study [PSI+] since nonsense suppression by [PSI+] allows cells to grow on synthetic medium lacking adenine (SC-ade) and prevents the build-up of adenine metabolites that would cause [psi-] cells (loss-of-[PSI+], soluble Sup35, no nonsense suppression) to turn red on rich media YPD. The AT3 strain, containing ade1-14, and its hsp104 variants were monitored for colony colour on YPD. As shown in , the parental AT3 (HSP104) strain is white (i.e., [PSI+]) and its Δhsp104 strain is red (i.e., [psi-]). The hsp104 mutants were either white, pink, or red (), indicating strong [PSI+], weak [PSI+] and [psi-], respectively. The profiles for each mutant, compared to wild-type Hsp104, are summarized in .
The loss-of-[PSI+] in hsp104 mutants that became red on YPD was further confirmed by tetrad analysis of HSP104/hsp104 heterozygous diploids made by crossing the hsp104 mutants with the wild-type [psi-] (NPK175) strain. All spores remained red on YPD media, showing that the hsp104 mutants could not transmit [PSI+] (data not shown). Hence we concluded that, of 58 hsp104 mutants made in this study, 18 did not affect [PSI+], nine weakly affected [PSI+], and 31 completely eliminated [PSI+].
Cellular level of mutated Hsp104 proteins.
To assess the possibility that Hsp104 mutations affected [PSI+] by altering levels of Hsp104 expression, we confirmed that Hsp104 levels were essentially the same for all alleles, except for the null allele Δhsp104, by Western blot (). Coomassie staining of the same protein blots confirmed the internal loading control of bulk-protein. Therefore, the affect on [PSI+] by these hsp104 derivatives was due to an altered activity of Hsp104.
Dominant negative hsp104 mutants.
When the hsp104 mutants (AT3 MATa) that caused loss-of-[PSI+] were mated with the HSP104 [PSI+] strain (K151 MATα), the HSP104/hsp104 heterozygotes showed different degrees of suppression of ade1-14, probably reflecting different efficiencies of prion propagation (data not shown). Upon sporulation, a 2:2 segregation of the [PSI+] element was observed in most cases, with the mutant hsp104 allele segregating with [psi-] (see typical example of Δhsp104 in ). Of interest, heterozygous diploids between wild-type HSP104 and G212D, G217V, G619V or G661D were red, and also produced four red spores (). These results suggest that these hsp104 alleles are dominant or semi-dominant and act to interfere with wild-type Hsp104 function. The dominant or semi-dominant phenotype of hsp104 mutants could be explained by either the formation of a nonfunctional Hsp104 hetero-hexamer or the interference with assembly of a functional hexamer.
Thermotolerance in hsp104 mutants.
Hsp104 is a heat-inducible protein required for thermotolerance and acts to resolubilize protein aggregates generated by severe stress, thereby allowing yeast to survive an otherwise lethal stress.Citation2,Citation3 All 58 hsp104 mutants constructed in this study were examined for thermotolerance. To measure thermotolerance, yeast cultures were exposed to 37°C for 60 minutes as a pretreatment to induce the heat-shock response then incubated at 50°C for 20 minutes. The extent of survival was determined from 5-fold serial dilutions spotted to YPD+ade plates at 30°C. Compared to the wild-type strain, about half of the hsp104 strains exhibited variably reduced thermotolerance (), and the results are summarized in .
Of 58 mutants, 29 showed no change in thermotolerance, 14 showed partial loss in thermotolerance, and 15 showed severe loss in thermotolerance. When combined with the [PSI+] phenotype, there appear two interesting classes: nine mutants (D184Y, A201V, Y257A, A298V, T317A, L462R, P557L, D704N, and T726I) are defective in [PSI+] but are normal in thermotolerance, while two mutants (L814S and L840Q) are normal in [PSI+] but are severely or partially defective in thermotolerance. Two mutants (D184N and C209Y) are defective in [PSI+] and also exhibit a reduction in thermotolerance (see ). These findings suggest that many domains and residues of Hsp104 are equally important for thermotolerance and [PSI+], but several residues, and hence specific structural unit(s), possess a distinct activity for prion propagation independent of thermotolerance.
Discussion
In previous studies, many hsp104 mutations have been isolated and characterized by genetic as well as biochemical means. A large portion of these Hsp104 mutants was plasmid, and not chromosomal-borne. It is known that the gene dosage of HSP104 influences the frequency of [PSI+] propagation since overexpression of Hsp104 eliminates [PSI+].Citation9 Hence, it is prudent to monitor possible effects of individual hsp104 mutations on [PSI+] using chromosomal alleles in the same strain background. Of additional importance is to gather separately reported or newly manipulated hsp104 mutations in the same isogenic mutant collection and investigate them under the same experimental conditions. From this viewpoint, the present collection should be useful as a comprehensive repertoire of hsp104 mutants to study Hsp104's remodeling activity.
Fifty-eight single hsp104 mutations were manipulated in the S. cerevisiae chromosome. Of these, 40 mutations are known in the literature including 21 in multiple amino acid substitution mutants (note that these single mutants have never been tested) (). Of these, only eleven have been examined for possible involvement in [PSI+].Citation7,Citation9,Citation28–Citation30,Citation32–Citation34,Citation38,Citation39 The remaining 18 novel mutations were newly engineered in this laboratory based on the predicted structure combined with available functional features of Hsp104.Citation31,Citation35–Citation37 Of the 58 mutants monitored, 31 were severely defective in [PSI+] propagation. Of these, eight partially lost thermotolerance, while 14 lost thermotolerance completely. Interestingly, the remaining nine mutants that lost [PSI+] (D184Y, A201V, Y257A, A298V, T317A, L462R, P557L, D704N, and T726I) remained thermotolerant (see ). Importantly, seven of these mutations are highly conserved residues from prokaryotes to eukaryotes (see ). In contrast, two hsp104 mutants (L814S and L840Q) showed the opposite phenotype, i.e., normal in [PSI+] but severely or partially defective in thermotolerance (see ). This is consistent with previous findings that L814S and L840Q derivatives are unable to complement the thermotolerance defect in yeast lacking hsp104 despite their ability to substitute for wild-type Hsp104 in in vitro refolding of model proteins and in [PSI+] propagation.Citation28 In that report, the authors surmised that exposure to extreme temperature inactivated these particular Hsp104 derivatives because of the observed temperaturedependent defects in ATPase activities.Citation28 It remains to be tested if L814S and L840Q show similar temperature-dependent defects in [PSI+] propagation. Nonetheless, the present findings suggest that Hsp104 can discriminate stress-induced protein aggregates and [PSI+] prion aggregates using Hsp104's specific domain(s) or residues. An alternative argument is that the observed differential effect of various hsp104 mutants may simply mean that both activities are based on the same mechanism, but prion propagation is more sensitive to subtle changes in activity, or in regulation of activity, than thermotolerance. The existence of two opposite types of hsp104 mutants, once established, will contribute to exclude this possibility. We became aware of a recent study that warrants mention. Doyle et al have reported that mixtures of ATP and ATP-γS affect Hsp104 remodeling activities differentially for stress-induced protein aggregates or prion amyloids.Citation40 This is in line with our argument that shared as well as distinct domains of Hsp104 are engaged in remodeling for different species of protein aggregated.
Given that Hsp104 is capable of discriminating species of protein aggregates, the straightforward explanation is that the mutated residues are directly (or indirectly) involved in the specific domain(s) of Hsp104 for substrate aggregate discrimination. The thermotolerance specific hsp104 mutations (L814S, L840Q) are consistent with the previous report that the C-terminal domain of Hsp104 is engaged in substrate binding.Citation39 Of the [PSI+]-specific hsp104 mutants, T317A was previously described to provide thermotolerance but is inactive in [PSI+] propagation.Citation32 Furthermore, our concurrent study of the genome-wide selection and characterization of loss-of-[PSI+] mutants independently uncovered three single mutants, L462R, P557L and D704N, as they eliminate [PSI+] but had no affect on thermotolerance.Citation27 In the literature, there is another [PSI+]-specific hsp104 mutation, D184Y, that is unaffected in thermotolerance but shows unstable propagation of [PSI+].Citation30 We have manipulated D184Y and D184N mutants and found, in our isogenic strain condition, that both of them completely eliminated [PSI+] and had little effect on thermotolerance (see ). These contrary results might be a result of strain difference. Other relevant mutations are H25Y, T160M and A503V. Although not tested in this study, H25Y and T160M are known to be thermotolerant and able to revert unstable propagation of [PSI+] in the SSA1-21 mutant, which shows [PSI+]-eliminating thermotolerant phenotype.Citation41,Citation42 It was also shown that the A503V mutation does not affect Hsp104's thermotolerance but is toxic to [PSI+] cells.Citation29,Citation43 In our hands, A503V did not affect Hsp104's thermotolerance as reported, nor was it toxic to [PSI+] cells, but rather partially antagonized [PSI+] (see ). These results may not be contrary to the previous report by Gokhale et alCitation43 since they observed that the severity of the toxic effect depended on the strain of [PSI+], and the [PSI+] strain in this study was independently isolated. Additionally, the different expression procedures, i.e., authentic chromosomal expression (this study) and plasmid-borne induction and overexpressionCitation29,Citation43 of the A503V protein, might account for, at least in part, the differences seen between these studies.
The tertiary structure of Thermus thermophilus ClpB protein, a bacterial homolog of Hsp104, consists of six domains, the N-terminal domain (NTD), nucleotide binding domain 1 (NBD1), D1-small domain, middle region (MR), NBD2, and C-terminal domain (CTD)/D2-small domain (; see also ).Citation6 The reconstituted Hsp104 hexamer forms a two-tiered ring structure ().Citation6 There is a ∼16 Å opening in the top ring (central pore) and six ∼10–30 Å wide channels on the lateral surface of the molecule (). The MR consists of an 85 Å long, mobile coiled coil that is located on the outside of the hexamer ().Citation6 It has been proposed that the central pore serves to thread stress-induced protein aggregates using a large coiled-coil motion of the MR driven by ATP hydrolysis.Citation6 In a concurrent study in this laboratory, we noticed that [PSI+]-eliminating thermotolerant mutants, T317A, L462R, P557L, D704N, are clustered around the lateral channel ().Citation27 Of the five newly identified additional members, A201V and T726I are also located within the vicinity of this channel (). The location of the additional Y257A and A298V mutations are uncertain due to the lack of the crystal structure of the relevant region. The D184Y mutation, which eliminates [PSI+] but remains thermotolerant, also maps within the lateral channel (). It is worth mentioning that these lateral channel mutations are located in NBD1 and NBD2 as well as in MR bridging NBD1 and NBD2 ( and ), suggesting the importance of nucleotide binding domains and the communication domain that constitute the lateral channel of Hsp104.
To our knowledge, there is no indication in the literature how the lateral channel plays a role in Hsp104's remodeling activity. One possibility suggests that Hsp104 uses the central pore to remodel stress-induced protein aggregates in general while the six lateral channels are specific to remodeling prion fiber, and this specific activity is impaired by the channel mutations. Considering the differential effect of mixtures of ATP and ATP-γS on Hsp104,Citation40 it is tempting to speculate that the lateral-channel remodeling activity is independent of ATP and ATP-γS, while the central-pore remodeling activity depends on ATP and ATP-γS. Alternatively, [PSI+]-eliminating thermotolerant hsp104 mutants might have increased ability to process [PSI+] to [psi-]. This is interesting because a [PSI+]-eliminating thermotolerant phenotype also appears upon overexpression of Hsp104.Citation9 Therefore, it might be possible that these mutations result in hyperactive Hsp104's, or Hsp104's with higher affinity or processivity for [PSI+], and thereby would also be thermotolerant. Additional possibilities including the inability to nucleate prion formationCitation24 cannot be excluded at present.
Finally, it is worth emphasizing that of the 58 hsp104 mutations that cover the entire region of Hsp104, [PSI+]-specific eliminating mutations are clustered within the lateral channel of the Hsp104 hexamer. Further study is necessary to elucidate the Hsp104 remodeling function and mechanism to discriminate substrate protein aggregates. The present hsp104 mutant repertoire will greatly contribute to this study.
Abbreviations
S. cerevisiae | = | Saccharomyces cerevisiae |
T. thermophilus | = | Thermus thermophilus |
AAA proteins | = | ATPases associated with various cellular activities |
NTD | = | N terminal domain |
NBD | = | nucleotide binding domain |
MR | = | middle region |
CTD | = | C terminal domain |
Figures and Tables
Figure 1 Hsp104 primary structure and location of mutations engineered in this study. The multi-domain structure of the Hsp104 monomer. The N-terminal domain (NTD), nucleotide-binding domains (NBD) 1 and 2, D1 small domain (D1sD), middle region (MR), and C-terminal domain (CTD) are indicated. The domain boundaries are according to Hsp104's alignment with the T. thermophilus ClpB sequence.Citation6 The hsp104 mutations are shown with their allele numbers, for example, A201V is an Ala-to-Val change at position 201.

Figure 2 The status of [PSI+] in hsp104 mutants is monitored by color assay of ade1-14 nonsense suppression. Haploid strains carrying the indicated hsp104 mutations and control wild-type [PSI+] strain (AT3) were incubated on YPD at 30°C for four days. The results are summarized in .
![Figure 2 The status of [PSI+] in hsp104 mutants is monitored by color assay of ade1-14 nonsense suppression. Haploid strains carrying the indicated hsp104 mutations and control wild-type [PSI+] strain (AT3) were incubated on YPD at 30°C for four days. The results are summarized in Table 3.](/cms/asset/28a04c0a-8d5f-4c44-9a0f-5c2fb5f01f4a/kprn_a_10904060_f0002.gif)
Figure 3 Hsp104-levels in mutant strains. Wild-type [psi-] (AT1), wild-type [PSI+] (AT3), Δhsp104 and 58 missense hsp104 mutants were cultured in YPD+ade liquid medium and harvested in mid-log phase. (Upper) Anti-Hsp104 immunoblotting. (Lower) Coomassie stained internal loading control.
![Figure 3 Hsp104-levels in mutant strains. Wild-type [psi-] (AT1), wild-type [PSI+] (AT3), Δhsp104 and 58 missense hsp104 mutants were cultured in YPD+ade liquid medium and harvested in mid-log phase. (Upper) Anti-Hsp104 immunoblotting. (Lower) Coomassie stained internal loading control.](/cms/asset/7db54225-b82f-4a88-98cf-ba1a635a17c8/kprn_a_10904060_f0003.gif)
Figure 4 Tetrad analysis of hsp104 mutant phenotypes monitored by nonsense suppression of the ade1-14 strain. Heterozygous diploids from matings between indicated hsp104 mutants and wild-type [PSI+] cells (NPK151) were sporulated and their progeny were incubated on YPD at 30°C for four days. Asterisks indicate the Leu+, hsp104 mutants.
![Figure 4 Tetrad analysis of hsp104 mutant phenotypes monitored by nonsense suppression of the ade1-14 strain. Heterozygous diploids from matings between indicated hsp104 mutants and wild-type [PSI+] cells (NPK151) were sporulated and their progeny were incubated on YPD at 30°C for four days. Asterisks indicate the Leu+, hsp104 mutants.](/cms/asset/3c36c5ff-e530-48ae-a8ce-0ea6feff2304/kprn_a_10904060_f0004.gif)
Figure 5 Effect of hsp104 mutations on thermotolerance. Mid-log phase cultures of the indicated strains were incubated at 37°C for one hour to induce the heat-shock response and were then incubated for a further 20 minutes at 50°C. The viability of heat-shocked cells (right) were compared to untreated cells (left) by five-fold serial dilutions on YPD+ade agar. Cells were incubated at 30°C for two days. The results are summarized in ().
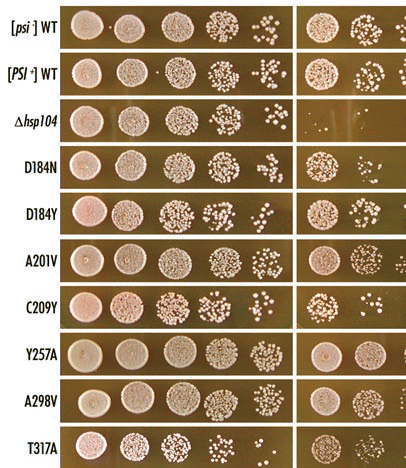
Figure 6 Structure of the ClpB/Hsp104 monomer and hexamer. (A) Ribbon drawing of T. thermophilus ClpB monomer (Protein Data Bank accession number 1QVR).Citation6 The figure shown was generated with the program PyMOL. Domain assignments were given by Lee et al.Citation6 Amino acid changes in hsp104 mutants (shown as grey, red or blue spheres) are assigned at the equivalent positions in ClpB (shown in parentheses). Red spheres show [PSI+]-eliminating thermotolerant mutations. Y257A and A298V mutations are not shown because these positions were not determined in the 3D structure of T. thermophilus ClpB. Blue spheres show hsp104 mutations that were able to propagate [PSI+] but lost thermotolerance. (B) Axial (left-top) and lateral (left-bottom) images of ClpB/Hsp104 hexamer (reprinted from Lee et al6 with permission from Elsevier). (Right) A close-up view of the lateral channel. The figure shown was generated with the program PyMOL. D184Y, A201V, T317A, L462R, P557L, D704N and T726I mutations, which remained thermotolerant but lost [PSI+] propagation, are indicated as red spheres. Parentheses indicate the equivalent positions in ClpB.
![Figure 6 Structure of the ClpB/Hsp104 monomer and hexamer. (A) Ribbon drawing of T. thermophilus ClpB monomer (Protein Data Bank accession number 1QVR).Citation6 The figure shown was generated with the program PyMOL. Domain assignments were given by Lee et al.Citation6 Amino acid changes in hsp104 mutants (shown as grey, red or blue spheres) are assigned at the equivalent positions in ClpB (shown in parentheses). Red spheres show [PSI+]-eliminating thermotolerant mutations. Y257A and A298V mutations are not shown because these positions were not determined in the 3D structure of T. thermophilus ClpB. Blue spheres show hsp104 mutations that were able to propagate [PSI+] but lost thermotolerance. (B) Axial (left-top) and lateral (left-bottom) images of ClpB/Hsp104 hexamer (reprinted from Lee et al6 with permission from Elsevier). (Right) A close-up view of the lateral channel. The figure shown was generated with the program PyMOL. D184Y, A201V, T317A, L462R, P557L, D704N and T726I mutations, which remained thermotolerant but lost [PSI+] propagation, are indicated as red spheres. Parentheses indicate the equivalent positions in ClpB.](/cms/asset/3a9103ed-ec37-411a-838a-a951dc41ac08/kprn_a_10904060_f0006.gif)
Table 1 Strains
Table 2 Primers used in this study
Table 3 Properties of hsp104 mutations
Acknowledgements
We thank Colin Crist for critical reading of the manuscript and valuable comments. This work was supported in part by grants from the following: The Ministry of Education, Sports, Culture, Science and Technology of Japan (MEXT); and the BSE Control Project of the Ministry of Agriculture, Forestry and Fisheries of Japan.
References
- Ogura T, Wilkinson AJ. AAA+ superfamily ATPases: Common structure-diverse function. Genes Cells 2001; 6:575 - 597
- Sanchez Y, Lindquist SL. HSP104 required for induced thermotolerance. Science 1990; 248:1112 - 1115
- Parsell DA, Kowal AS, Singer MA, Lindquist S. Protein disaggregation mediated by heat-shock protein Hsp104. Nature 1994; 372:475 - 478
- Glover JR, Lindquist S. Hsp104, Hsp70, and Hsp40: A novel chaperone system that rescues previously aggregated proteins. Cell 1998; 94:73 - 82
- Schirmer EC, Glover JR, Singer MA, Lindquist S. HSP100/Clp proteins: A common mechanism explains diverse functions. Trends Biochem Sci 1996; 21:289 - 296
- Lee S, Sowa ME, Watanabe YH, Sigler PB, Chiu W, Yoshida M, Tsai FT. The structure of ClpB: A molecular chaperone that rescues proteins from an aggregated state. Cell 2003; 115:229 - 240
- Lum R, Tkach JM, Vierling E, Glover JR. Evidence for an unfolding/threading mechanism for protein disaggregation by Saccharomyces cerevisiae Hsp104. J Biol Chem 2004; 279:29139 - 29146
- Weibezahn J, Tessarz P, Schlieker C, Zahn R, Maglica Z, Lee S, Zentgraf H, Weber-Ban EU, Dougan DA, Tsai FT, Mogk A, Bukau B. Thermotolerance requires refolding of aggregated proteins by substrate translocation through the central pore of ClpB. Cell 2004; 119:653 - 665
- Chernoff YO, Lindquist SL, Ono B, Inge-Vechtomov SG, Liebman SW. Role of the chaperone protein Hsp104 in propagation of the yeast prion-like factor [psi+]. Science 1995; 268:880 - 884
- Moriyama H, Edskes HK, Wickner RB. [URE3] prion propagation in Saccharomyces cerevisiae: Requirement for chaperone Hsp104 and curing by overexpressed chaperone Ydj1p. Mol Cell Biol 2000; 20:8916 - 8922
- Sondheimer N, Lindquist S. Rnq1: An epigenetic modifier of protein function in yeast. Mol Cell 2000; 5:163 - 172
- Ness F, Ferreira P, Cox BS, Tuite MF. Guanidine hydrochloride inhibits the generation of prion ”seeds“ but not prion protein aggregation in yeast. Mol Cell Biol 2002; 22:5593 - 5605
- Cox BS. ψ, a cytoplasmic suppressor of super-suppressor in yeast. Heredity 1965; 20:505 - 521
- Wickner RB. [URE3] as an altered URE2 protein: Evidence for a prion analog in Saccharomyces cerevisiae. Science 1994; 264:566 - 569
- Sparrer HE, Santoso A, Szoka FC Jr, Weissman JS. Evidence for the prion hypothesis: Induction of the yeast [PSI+] factor by in vitro- converted Sup35 protein. Science 2000; 289:595 - 599
- King CY, Diaz-Avalos R. Protein-only transmission of three yeast prion strains. Nature 2004; 428:319 - 323
- Tanaka M, Chien P, Naber N, Cooke R, Weissman JS. Conformational variations in an infectious protein determine prion strain differences. Nature 2004; 428:323 - 328
- Stansfield I, Jones KM, Kushnirov VV, Dagkesamanskaya AR, Poznyakovski AI, Paushkin SV, Nierras CR, Cox BS, Ter-Avanesyan MD, Tuite MF. The products of the SUP45 (eRF1) and SUP35 genes interact to mediate translation termination in Saccharomyces cerevisiae. EMBO J 1995; 14:4365 - 4373
- Zhouravleva G, Frolova L, Le Goff X, Le Guellec R, Inge-Vechtomov S, Kisselev L, Philippe M. Termination of translation in eukaryotes is governed by two interacting polypeptide chain release factors, eRF1 and eRF3. EMBO J 1995; 14:4065 - 4072
- Ehrenberg M, Hauryliuk V, Crist CG, Nakamura Y. Mathews MB, Sonenberg N, Hershey JWB. Translation termination, prion [PSI+], and ribosome recycling. Translational Control in Biology and Medicine 2007; New York Cold Spring Harbor Laboratory Press 173 - 196
- Liebman SW, Sherman F. Extrachromosomal ψ+ determinant suppresses nonsense mutations in yeast. J Bacteriol 1979; 139:1068 - 1071
- Patino MM, Liu JJ, Glover JR, Lindquist S. Support for the prion hypothesis for inheritance of a phenotypic trait in yeast. Science 1996; 273:622 - 626
- Paushkin SV, Kushnirov VV, Smirnov VN, Ter-Avanesyan MD. Propagation of the yeast prion-like [psi+] determinant is mediated by oligomerization of the SUP35-encoded polypeptide chain release factor. EMBO J 1996; 15:3127 - 3134
- Shorter J, Lindquist S. Destruction or potentiation of different prions catalyzed by similar Hsp104 remodeling activities. Mol Cell 2006; 23:425 - 438
- True HL. The battle of the fold: Chaperones take on prions. Trends Genet 2006; 22:110 - 117
- Crist CG, Nakayashiki T, Kurahashi H, Nakamura Y. [PHI+], a novel Sup35-prion variant propagated with non-Gln/Asn oligopeptide repeats in the absence of the chaperone protein Hsp104. Genes Cells 2003; 8:603 - 618
- Kurahashi H, Nakamura Y. Channel mutations in Hsp104 hexamer distinctively affect thermotolerance and prion-specific propagation. Mol Microbiol 2007; 63:1669 - 1683
- Tkach JM, Glover JR. Amino acid substitutions in the C-terminal AAA+ module of Hsp104 prevent substrate recognition by disrupting oligomerization and cause high temperature inactivation. J Biol Chem 2004; 279:35692 - 35701
- Schirmer EC, Homann OR, Kowal AS, Lindquist S. Dominant gain-of-function mutations in Hsp104p reveal crucial roles for the middle region. Mol Biol Cell 2004; 15:2061 - 2072
- Jung G, Jones G, Masison DC. Amino acid residue 184 of yeast Hsp104 chaperone is critical for prion-curing by guanidine, prion propagation, and thermotolerance. Proc Natl Acad Sci USA 2002; 99:9936 - 9941
- Mogk A, Schlieker C, Strub C, Rist W, Weibezahn J, Bukau B. Roles of individual domains and conserved motifs of the AAA+ chaperone ClpB in oligomerization, ATP hydrolysis, and chaperone activity. J Biol Chem 2003; 278:17615 - 17624
- Hattendorf DA, Lindquist SL. Cooperative kinetics of both Hsp104 ATPase domains and interdomain communication revealed by AAA sensor-1 mutants. EMBO J 2002; 21:12 - 21
- Hattendorf DA, Lindquist SL. Analysis of the AAA sensor-2 motif in the C-terminal ATPase domain of Hsp104 with a site-specific fluorescent probe of nucleotide binding. Proc Natl Acad Sci USA 2002; 99:2732 - 2737
- Parsell DA, Sanchez Y, Stitzel JD, Lindquist S. Hsp104 is a highly conserved protein with two essential nucleotide-binding sites. Nature 1991; 353:270 - 273
- Liu Z, Tek V, Akoev V, Zolkiewski M. Conserved amino acid residues within the amino-terminal domain of ClpB are essential for the chaperone activity. J Mol Biol 2002; 321:111 - 120
- Tanaka N, Tani Y, Hattori H, Tada T, Kunugi S. Interaction of the N-terminal domain of Escherichia coli heat-shock protein ClpB and protein aggregates during chaperone activity. Protein Sci 2004; 13:3214 - 3221
- Barnett ME, Zolkiewski M. Site-directed mutagenesis of conserved charged amino acid residues in ClpB from Escherichia coli. Biochemistry 2002; 41:11277 - 11283
- Schirmer EC, Queitsch C, Kowal AS, Parsell DA, Lindquist S. The ATPase activity of Hsp104, effects of environmental conditions and mutations. J Biol Chem 1998; 273:15546 - 15552
- Cashikar AG, Schirmer EC, Hattendorf DA, Glover JR, Ramakrishnan MS, Ware DM, Lindquist SL. Defining a pathway of communication from the C-terminal peptide binding domain to the N-terminal ATPase domain in a AAA protein. Mol Cell 2002; 9:751 - 760
- Doyle SM, Shorter J, Zolkiewski M, Hoskins JR, Lindquist S, Wickner S. Asymmetric deceleration of ClpB or Hsp104 ATPase activity unleashes protein-remodeling activity. Nat Struct Mol Biol 2007; 14:114 - 122
- Hung GC, Masison DC. N-terminal domain of yeast Hsp104 chaperone is dispensable for thermotolerance and prion propagation but necessary for curing prions by Hsp104 overexpression. Genetics 2006; 173:611 - 620
- Jung G, Jones G, Wegrzyn RD, Masison DC. A role for cytosolic Hsp70 in yeast [PSI+] prion propagation and [PSI+] as a cellular stress. Genetics 2000; 156:559 - 570
- Gokhale KC, Newnam GP, Sherman MY, Chernoff YO. Modulation of prion-dependent polyglutamine aggregation and toxicity by chaperone proteins in the yeast model. J Biol Chem 2005; 280:22809 - 22818