Abstract
The yeast prion phenomenon is very widespread and mounting evidence suggests that it has an impact on cellular regulatory mechanisms related to phenotypic responses to changing environments. Studying the aggregation patterns of prion amyloids during different stages of the prion life cycle is a first key step to understand major principles of how and where cells generate, organize and turn-over prion aggregates. The induction of the [PSI+] state involves the actin cytoskeleton and quality control compartments such as the Insoluble Protein Deposit (IPOD). An initially unstable transitional induction state can be visualized by overexpression of the prion determinant and displays characteristic large ring- and ribbon-shaped aggregates consisting of poorly fragmented bundles of very long prion fibrils. In the mature prion state, the aggregation pattern is characterized by highly fragmented, shorter prion fibrils that form aggregates, which can be visualized through tagging with fluorescent proteins. The number of aggregates formed varies, ranging from a single large aggregate at the IPOD to multiple smaller ones, depending on several parameters discussed. Aggregate units below the resolution of light microscopy that are detectable by fluorescence correlation spectroscopy are in equilibrium with larger aggregates in this stage and can mediate faithful inheritance of the prion state. Loss of the prion state is often characterized by reduced fragmentation of prion fibrils and fewer, larger aggregates.
Introduction
The existence of prions in yeast has been recognized 17 years ago when it was proposed that the yeast transcriptional co-repressor Ure2p can exist in at least two different stable conformations, one soluble, cellular form that is active as a repressor through binding and sequestration of two transcriptional activators, and an insoluble conformation that is inactive.Citation1 This alternative conformation has the unusual ability to template the conversion of proteins of the same type/amino acid sequence into it’s own prion-like conformation in a dominant fashion, just like it was proposed for the mammlian protein Prp that is associated with fatal neurodegenerative prion diseases.Citation2 A rapidly growing research interest in this phenomenon has not only proven the hypothesis correct for yeast, but has aided to a great extend the better understanding of the mammalian prion phenomenon as well.
Currently, at least seven different, functionally and sequence-wise unrelated proteins have been proposed to act as a prion in Saccharomyces cerevisiae, with many more potential prions being possible ([MOT3+],[OCT+], [SWI+], [URE3], [PSI+], [ISP+], [RNQ+]).Citation3-Citation6 The biological meaning of the prion phenomenon in yeast is still controversially discussed, but it is striking that many of the prion-like proteins function in global gene regulation at either transcriptional or post-translational level. This raises the possibility that conversion of prion proteins into their prion conformation with an associated partial loss-of function or gain-of function phenotype could co-regulate a wide range of cellular functions.Citation4-Citation6 Among the three best-studied prions [RNQ+], [PSI+] and [URE3], only [RNQ+] was detected in some of ~70 tested natural yeast populations, which could mean that there is strong selective pressure against prions.Citation7 In fact, the majority phenotypes associated with [PSI+] can be detrimental, but ~25% of them can be advantageous.Citation8-Citation10 Furthermore, the conservedCitation11 ability to adopt a prion conformation and associated new phenotype,Citation4-Citation6 and the responsiveness of the [PSI+] system to environmental stressesCitation12-Citation15 supports the hypothesis that the [PSI+] prion is an evolutionary capacitor that facilitates adaptation of yeast to fluctuating environments and might impact the evolution of new traits.Citation8,Citation9,Citation16,Citation17 Such a function may not be limited to the [PSI+] prion, but could apply to other yeast prions.Citation4,Citation5,Citation18
As for their mammalian counterparts, yeast prions can exist in different yet stable prion strains or variants. They were explained by slightly different conformations of the prion protein in the prion state and the associated biophysical properties, and cause variation of the phenotype associated with the prion.Citation19-Citation31
This mini-review will focus mainly on the [PSI+] prion as the best studied example and only refer to other yeast prions in some particular examples.
Monitoring the Prion Status
For a prion to faithfully, but only metastably propagate, the rates of conversion from the cellular conformation to the prion form of a given protein, the rates of splitting of prion aggregates into smaller transmissible units referred to as propagons,Citation32 and their transfer to daughter cells has to keep pace with the speed of cell growth and division,Citation33 which itself is not a constant, but varies depending on the environmental conditions. To study these parameters and their complex interplay, the prion status of a cell must be monitored over time in a dynamic way. To meet these requirements, mainly two experimental strategies have been used in the past, either to monitor the phenotype associated with the prion or the conformational state of the prion protein itself.
Whereas many robust experimental methods exist to monitor the [PSI+] phenotype,Citation34-Citation37 the assessment of the phenotypes associated with other yeast prions is often more complicated.Citation5,Citation6
Alternatively to the phenotype associated with the prion, the conformational state of the protein determinant can also allow conclusions about the prion status of the cell. Although different methods have been established most comprehensively for the [PSI+] prion and [URE3], they are usually also more commonly applicable to other yeast prions. However, the biggest draw-back with some of these methods is that they depict an incomplete picture of the conformational state of a protein, because they can either not distinguish between amorphous and amyloidogenic aggregates, or they can do so, but cannot be used in living cells.Citation34-Citation37 Therefore, a combination of various different methods, often additionally supported by phenotypic tests, is necessary to clearly reveal the conformational state of a prion protein in vivo. The most commonly used methods include 3 major experimental approaches: 1) Monitoring changes in the conformation of the prion protein by differences in solubility and protease resistanceCitation38-Citation41; 2) Isolation and detection of amyloid-like conformations in an agarose based method called SDD-AGE (semi-denaturing detergent-agarose gel electrophoresis)Citation42,Citation43 or in filter retardation assays.Citation44 Herein, cell lysates are treated with an SDS buffer to solubilize most macromolecular complexes and aggregates, but leaving amyloid like aggregates intact. Samples are then separated in an agarose gel and transferred onto blotting membranes for SDD-AGE, or transferred directly onto cellulose acetate with a vacuum device for the filter retardation assay. The final step for both methods is antibody-detection; 3) Visualizing prion proteins with different microscopy-based techniques. Those include a) fluorescence microscopy using antibodies against the prion determining proteinCitation45-Citation47 or b) fluorescently labeled prion proteins or truncations thereof (the prion determining region fused to fluorescent proteins)Citation40,Citation3,Citation38,Citation41,Citation48-Citation50, c) fluorescence correlation spectroscopy (FCS), which has a much higher size resolution and allows to judge aggregated units of the Sup35 protein that are below the resolution of fluorescence microscopy,Citation51 d) amyloid staining of prion aggregates with fluorescent dyes that bind amyloid in vivo, followed by microscopic detection,Citation47 and e) electron microscopy, that can be used to visualize the typical fibrillar structure of amyloid like aggregates, either in vitroCitation41,Citation52-Citation55 or in situ.Citation56-Citation58
The Conformational State of [PSI+]-Like Prions In Vivo
The characterization of the prion conformation especially in living cells has been done most comprehensively for the [PSI+] prion. Through the combination of different methods, a picture of the cellular conformation of the prion aggregates starts to emerge:
In the prion free state, the Sup35 protein is soluble, but becomes insoluble in centrifugation assays when the cells adopt the [PSI+] state. Furthermore, the Sup35 protein isolated from [PSI+] cells was more proteinaseK resistant than from [psi-] cells ,(Citation38,Citation39) which predicted conformational alterations of the protein in the prion conformation.
To visualize the aggregated protein in [PSI+] cells, a reporter consisting of the prion determining N- and M-domains of Sup35 (NM) fused with GFP was expressed transiently in [psi-]- and [PSI+] cells. Whereas the GFP fluorescence was diffuse in prion-free cells, it aggregated and accumulated into several fluorescent foci in [PSI+] cells.Citation38 While the conformational nature of the aggregates in vivo was initially unclear, their subsequent intense characterization clarified this open question. For instances, using the amyloid staining dye thioflavinS in vivo revealed that cells expressing a tagged NM-domain of Sup35 formed in fact thioflavinS positive aggregates.Citation47 SDD-AGE also predicted an amyloid-like structure of [PSI+] aggregates in vivo.Citation42,Citation43
To further unravel the structure of [PSI+]-aggregates (and other yeast prions), it was shown, initially in vitro, that purified prion proteins or just their prion determining domains were able to form characteristic amyloid fibers with a diameter between ~10 – 25 nm visible in electron microscopy.Citation52,Citation55,Citation59,Citation60 To proof that these in vitro generated [PSI+] fibers (and [URE3], [RNQ+] and [SWI+] prion fibers) corresponded to the infectious prion conformation, they were directly transformed into yeast cells devoid of the prion, where the fibers were able to induce the [PSI+] phenotype in the endogenous Sup35 protein.Citation25,Citation26,Citation61-Citation63 Moreover, these fiber transformations delivered the formal proof of the protein-only hypothesis for yeast prions.
Isolation of rod-like structures from [PSI+] cells that resembled fibrous structures in EM gave the fist hints for the existence of amyloid-like fibers of Sup35 in [PSI+] cells in vivo.Citation64 Additional strong evidence came from in situ electron microscopy, initially with Ure2 overexpressed in [URE3] cells,Citation56 and later in [PSI+] cells where overexpression of NM-GFP allowed to visualize fibrillar structures formed by NM-GFP within yeast cells.Citation57,Citation58 These NM-GFP fibrils resembled in their dimensions those that formed in vitro from the purified Sup35 protein or the corresponding prion domain alone. Amyloid-like conformations are anticipated for many other yeast prions as well.(Citation3,Citation56,Citation61-Citation63)
Aggregation Patterns of the [PSI+] Prion
When using fluorescently labeled proteins to visualize prion aggregates and the prion state within cells, it became obvious very early on that there is not a single, unique aggregation pattern typical for the [PSI+] state, but many different ones, depending on the individual experimental conditions used ().
Figure 1. Different aggregation patterns of the fluorescently tagged Sup35 protein or the prion determining NM-region. (A, B) GFP was fused between the N and M domain of Sup35 and replaced with the endogenous SUP35 locus, expressed from endogenous Sup35 promoter, either in [psi-] (A) or [PSI+])B); (C-H) Fluorescence pattern of NM-GFP in cells deleted for the endogenous NM-domain in SUP35, expressed at different levels; (C) NM-GFP under control of the SUP35 promoter in the [prion-] state. (D, E) Clonal isolates of cells expressing NM-GFP from the SUP35 promoter after mating with a [PSI+] strain, sporulation and tetrad dissection; (F) [RNQ+] cells expressing NM-GFP constitutively from the GPD promoter, immediately after transformation with the expression construct; (G) [RNQ+] cells expressing NM-GFP constitutively from the GPD promoter, ~3–5 d after transformation with the construct, transitional induction state; (H) [RNQ+] cells expressing NM-GFP constitutively from the GPD promoter, ~10 d after transformation with the construct (mature prion state)Citation58; (I, J) Electron microscopy of ring and dot cells as in G and H, after fixation, staining with uranyl acetate and ultrathin-sectioning; (I) ring cell, (J) dot cell.Citation58
![Figure 1. Different aggregation patterns of the fluorescently tagged Sup35 protein or the prion determining NM-region. (A, B) GFP was fused between the N and M domain of Sup35 and replaced with the endogenous SUP35 locus, expressed from endogenous Sup35 promoter, either in [psi-] (A) or [PSI+])B); (C-H) Fluorescence pattern of NM-GFP in cells deleted for the endogenous NM-domain in SUP35, expressed at different levels; (C) NM-GFP under control of the SUP35 promoter in the [prion-] state. (D, E) Clonal isolates of cells expressing NM-GFP from the SUP35 promoter after mating with a [PSI+] strain, sporulation and tetrad dissection; (F) [RNQ+] cells expressing NM-GFP constitutively from the GPD promoter, immediately after transformation with the expression construct; (G) [RNQ+] cells expressing NM-GFP constitutively from the GPD promoter, ~3–5 d after transformation with the construct, transitional induction state; (H) [RNQ+] cells expressing NM-GFP constitutively from the GPD promoter, ~10 d after transformation with the construct (mature prion state)Citation58; (I, J) Electron microscopy of ring and dot cells as in G and H, after fixation, staining with uranyl acetate and ultrathin-sectioning; (I) ring cell, (J) dot cell.Citation58](/cms/asset/1ba944e5-f71a-449f-93a4-6611deae529c/kprn_a_10918986_f0001.gif)
Two major kinds of constructs that are commonly used to visualize [PSI+] aggregates are fusions of just the prion-determining NM-domain of Sup35 to fluorescent proteins (NM-GFP)Citation38,Citation45,Citation46,Citation51,Citation58,Citation65,Citation66 or a functional fusion of the full length protein with GFP, where the GFP moiety is incorporated between the N-terminal and the middle domain of SUP35 (N-GFP-MC).Citation67-Citation70 In the prion state, the latter type of constructs tends to form very many tiny, sometimes hardly visible aggregatesCitation68 to multiple small aggregatesCitation67 (). In the prion state, NM-GFP fusions typically range from multiple small aggregates () over a few larger ones () to only one single large visible aggregateCitation45,Citation46,Citation51,Citation58,Citation65 ().
Even the aggregation patterns within one single construct can be dramatically different. Not only a change in the expression levels, but also manipulating factors that influence prion biogenesis, often have a dramatic effect on the aggregation behavior. For the two most commonly used constructs, NM-GFP and N-GFP-MC, a tendency is that higher expression levels of the same construct result in fewer, but bigger aggregates (compare ; refs.Citation71-Citation73). In case of manipulations that interfere with prion propagation, it was often observed that a decrease in the fidelity of prion propagation (for example curing) also results in larger and fewer aggregates,Citation42,Citation45,Citation68-Citation70,Citation74-Citation76 but this trend cannot be generalized under all conditions.Citation77,Citation78 Additional factors that have been reported to influence the aggregation patterns are growth conditions such as starvation or stationary phaseCitation46,Citation68 or stress conditions.Citation71,Citation79 For the [PSI+] and [RNQ+] prions, it was even established that the same construct can exist in various aggregation patterns that are influenced by the particular prion strain or variant. Different variants of [PSI+] with a different strength of the read through phenotype display differently sized amyloid aggregates in SDDAGE.Citation30,Citation31,Citation42 Moreover, for the [RNQ+] prion, it was shown that different variants of the prion have even very different aggregation patterns when the reporter Rnq1-GFP is expressed, a multiple-dot fluorescence pattern or a single-dot pattern.Citation80
Aggregation Patterns During De Novo Formation of the [PSI+] Prion
The spontaneous frequency of prion induction is very low and ranges at about 6 × 10-7.Citation17,Citation81,Citation82 Therefore, it is difficult to observe this event in a living cell under normal physiological conditions. However, overexpression of Sup35, it’s prion domain alone or fusions thereof leads to a very frequent induction of the [PSI+] stateCitation14,Citation83-Citation85 and can be observed under the microscope. Thus, to study the events occurring in a cell during de novo induction of the [PSI+] prion, various different approaches use overexpression of a prion domain containing “inducer” construct.Citation46,Citation58,Citation65,Citation66,Citation86 One aspect that has to be considered in such studies is that prolonged overexpression of the prion domain of Sup35 in the presence of wild type [PSI+] is toxic, due to excessive sequestration of the essential Sup35 protein or its partner protein Sup45.Citation14,Citation84,Citation85,Citation87,Citation88 One strategy to avoid this problem is to only transiently overexpress the inducer construct in [psi-] cells and monitor the prion status of the cell of interest or its progeny by other means or with GFP fusion constructs at lower and thereby non-toxic expression levels.Citation46,Citation65,Citation66 A second strategy is to delete the endogenous prion domain from the genomic SUP35 locus. This eliminates the toxicity of NM overexpression and therefore allows to maintain the higher expression levels and continuously follow NM-GFP aggregation over long periods of time. For the latter strategy, it was confirmed that the NM-GFP construct expressed at high levels itself is the prion that closely resembles the prion properties of [PSI+].Citation58 Both strategies revealed that overexpression of Sup35 or NM-GFP fusions resulted in the emergence of large ring-, rod or ribbon-like aggregatesCitation46,Citation58,Citation65,Citation66 (compare and ). Cultures with these ring- and ribbon like aggregates transitioned very frequently to the [PRION+] state with its typical aggregation patterns, either multiple small aggregates when the inducer construct was turned off and the prion state was monitored with a different construct at endogenous expression levelsCitation66 (Fig. One B), or as 1 single large dot aggregate when expression levels of the NM-GFP construct remained highCitation46,Citation51,Citation58,Citation65 (). When the cellular localization of the rings was further characterized,Citation65,Citation66 they were observed at two different sites, either in the cell periphery or surrounding the vacuole. Peripheral rings were commonly observed during transient overexpression of NM-GFP, whereas they transitioned to vacuolar rings after the overexpression construct was turned off.Citation66 These two cellular locations could represent two subsequent states in the prion induction process.
Figure 2. Model for aggregate organization in a cell (A) during induction of the NM-GFP prion state or (B) during propagagtion of the mature prion state. For details, see text section “Model for cellular organization of prion aggregates during induction and in the mature prion state.”
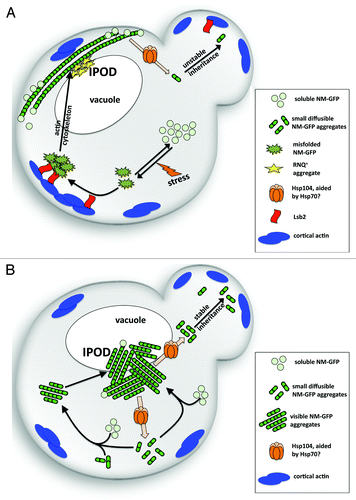
It was confirmed that the rings were no artifact of the GFP moiety in the fusion, as they were also observed with immunofluorescence methods when wild type Sup35 protein was overexpressed.Citation46 At continuously high expression levels, a mother cell with a ring most commonly kept the ring aggregate for herself during division, but the daughter cell often formed a ring or ribbon-like aggregate initiating from a small fluorescent focus that slowly grew out to form a ring. Similar to wild type [PSI+],Citation89 the initial formation of the rings required the presence of the [PSI+] inducer prion [RNQ+], but the propagation of the ring state, once emerged, occurred also in the absence of [RNQ+]. Thus, the ring state can be inherited for up to several generations, although much less stable as compared with the mature prion, because a decent number of daughter cells from ring mothers lost the aggregation state.Citation58
The molecular nature of these rings is still not entirely clear, but several findings in the field have started to unravel this curiosity. Electron microscopy revealed that the rings and ribbons consist of NM-GFP fibers indistinguishable from those found in dot-shaped aggregates of NM-GFP in [PSI+] cells.Citation57,Citation58 The only obvious difference between the fibers in the rings and dots characteristic for the mature [PRION+] state was that they were much longer in rings. In fact, inhibiting the fiber fragmentation activity of Hsp104 in cells possessing highly fragmented fibers in dots caused the progeny to produce rings again.Citation46,Citation58,Citation77 Consistently, lysates prepared from these ring cells using glass beads that could shear fibrils, induced the same strong [PSI+] variant with the same frequency as lysates from cells harboring dots,Citation58 when assayed in a fiber transformation test.Citation25,Citation26
An initially unstable intermediate or poorly heritable amyloids during prion induction by overexpression of the prion determinant have been observed for different prions before.Citation89-Citation93 Furthermore, similar ring-like intermediates have been visualized for other prions during the induced de novo prion formation process.Citation3,Citation66,Citation91,Citation94 Therefore, an unstable intermediate may be common to the prion induction process, possibly as a result of initially low amounts of prion seeds, larger size of fibrillar units and/or an initially low fragmentation activity in the cell (). However, detection of such a state in cells with naturally emerging [PSI+] with its low spontaneous induction frequency and the lower expressions levels of Sup35 seems technically very challenging, if possible.
Prion Deposition Sites in Yeast
The diversity of prion aggregation patterns means that there is no particular cellular localization or organization type of the prion aggregates required at all times for faithful prion biogenesis. Does this mean that prion aggregates are just randomly distributed in the cytoplasm of the cell, or are there nonetheless specific cellular sites involved with prion biogenesis? Recent evidence suggests that there are preferential perivacuolar sites in the cell where prion aggregates can accumulate.Citation58,Citation66,Citation86,Citation95 One of those sites was defined as a quality control site termed IPOD (Insoluble Protein Deposit) where terminally aggregated proteins are deposited.Citation95 It is a perivacuolar site close to the “pre-autophagosomal structure (PAS)” where the cell initiates autophagy.Citation96,Citation97 A typical marker for the IPOD site is Atg8, a protein crucial for macroautophagy that is commonly used as autophagic marker. GFP fused to the N-terminus of Atg8 forms 1 visible fluorescent focus next to the vacuole at the PASCitation97 and partially co-localizes with different IPOD substrates. Remarkably, aggregated polyQ-expansion mutants of huntingtin exon 1 fragment (Htt-103Q) as well as the prion protein determinants Ure2 and Rnq1 have been identified at this quality control compartment.Citation95 These two substrates can also be used as IPOD markers. Moreover, the dots that form when NM-GFP, expressed at high levels, is in the mature prion state, localize to this cellular site.Citation58 Electron microscopic images of such cells with dots confirmed the amyloidogenic fibrous structure of NM-GFP present at the IPOD. The fibrils were arranged in a higher level of order as stacked bundles of fibers, or in a honeycomb-like patternCitation57,Citation58 ().
When NM-GFP is expressed at levels of wild type Sup35, e.g., under control of the Sup35 promoter, it forms multiple smaller aggregates (compare ), whereas the IPOD is one single perivacuolar site.Citation95 Under these conditions, it is unknown whether one of the multiple foci of NM-GFP represents an IPOD localization and is in equilibrium with additional peripheral aggregates, or if prion aggregates are only deposited at the IPOD when the prion determinant is expressed at high levels, as a possible overflow mechanism of the protein quality control system that handles prion aggregates. However, several observations favor a role of the IPOD in accumulation of prion aggregates also under non-overexpression conditions: 1) when NM-GFP is transiently overexpressed in [PSI+] cells with an inducible promoter, the first visible fluorescent aggregates after induction appear as the single dot-shaped aggregate adjacent to the vacuole (see refs. Citation46,Citation51,Citation65; Tyedmers J., unpublished observation), arguing that some prion seeds of the naturally occurring [PSI+] are likely present at this site already, which start to incorporate newly synthesized NM-GFP molecules or freshly converted protein. 2) when isolating NM-GFP from cells carrying the characteristic NM-GFP dot at the IPOD, one of the co-precipitating factors was endogenous Rnq1, suggesting that RNQ aggregates are naturally present at this compartment as well.Citation58,Citation95 3) overexpression of GFP-fusions of various prion-like Q/N-rich domains from different proteins as well as the non Q/N-rich HET-s prion domain from the filamentous fungus Podospora anserina in S. cerevisiae also leads to a frequent occurrence of ring- and ribbon-like structures as well as single dots, reminiscent of the NM-GFP aggregates.Citation3,Citation91,Citation94
These observations make it tempting to speculate that cells have indeed common distinct cellular sites for deposition of various different prion- and other potentially amyloid-like proteins, and the deposits are in equilibrium with liberation of portions of the prion aggregates by Hsp104 (see below).Citation51,Citation57,Citation58,Citation70,Citation95 What might be the purpose of depositing aggregates at a particular cellular site? It was proposed that it could facilitate cross-seeding eventsCitation58,Citation86 occurring during de novo prion formation between different prions and amyloids such as [RNQ+] and [PSI+].Citation91,Citation92,Citation98,Citation99 In the case of NM-GFP, this hypothesis would be supported by the observation that even the prion induction intermediates, the rings and ribbons, that initiate as small foci and elongate at both ends to form the ring, intersect with the IPODCitation58 as if they initiated there. This would be consistent with the observation that NM-GFP rings appear at the cell periphery upon induction of overexpressionCitation66 if the IPOD was located in proximity to the cell periphery in these cells (compare ). An alternative possibility is that the initial prion conversion reaction takes place elsewhere, e.g., at the cell periphery in association with the cortical actin cytoskeleton, and amyloid aggregates are then deposited at the IPOD after prion induction.Citation66,Citation86 Next to serving as a possible location involved in prion generation, it is a striking feature that the IPOD localizes next to the site where the cell initiates autophagy, the PAS.Citation58,Citation95 Such a localization would be consistent with turn-over of aggregates by autophagy, very similar to what was observed for amyloidogenic aggregates in mammals.Citation100 However, there is no experimental evidence for this so far. Another hypothesis is that the IPOD compartment is an ancient structure that aids to maintain damaged proteins in the mother cells to spare progeny from accumulating damage, because the IPOD resided in mother cells during cell division.Citation95 This would be consistent with a series of studies suggesting that protein aggregates may be senescence factors that are retained in mother cells during cell division to rejuvenate the progeny.Citation101-Citation104 In fact, single large NM-GFP prion dots observed at higher expression levels of NM-GFP stay selectively in the mother cell during cell division.Citation58 Nonetheless, those aggregates that can act as propagonsCitation32 are faithfully inherited to daughter cells.Citation51,Citation58 Thus, a sufficient amount of aggregates can escape a potential retention mechanism and mediate faithful prion propagation, for example because their size may be below a threshold for efficient transmission.Citation70,Citation73
Proteins Interfering with Prion Aggregation Patterns
Several proteins are known to interfere with prion formation or propagation. Major functional categories of those proteins include other aggregation prone proteins, for example other prions like [RNQ+],Citation41 that influence the appearance of [PSI+],Citation19,Citation89,Citation91,Citation92,Citation98 molecular chaperones,Citation33,Citation36,Citation105-Citation108 the actin cytoskeletonCitation65,Citation66,Citation86,Citation109 and the ubiquitin-proteasome pathway,Citation14,Citation110,Citation111 in addition to several less well characterized factors.Citation14,Citation109,Citation112 Since the functional role of many modulators is not clear to date, it will be focused here on the better studied ones.
The most crucial factor for prion biogenesis is the molecular chaperone Hsp104.Citation113 It is required for faithful propagation of all confirmed yeast prions identified to date.Citation3-Citation6,Citation20 The exact molecular role of Hsp104 on prion biogenesis, especially in vivo, is still under debate (for more detailed reviews see for exampleCitation78,Citation108,Citation114,Citation115), but it is undoubted that it’s function in fiber severing plays an essential role for prion propagationCitation39,Citation70,Citation74,Citation113,Citation116,Citation117,Citation42,Citation45. Not surprisingly, modulating the activity of Hsp104 or the affinity of the substrate for Hsp104 results in dramatic changes in the patterns of aggregation.Citation42,Citation45,Citation46,Citation69,Citation70,Citation76 The trend is that the aggregate size increases the less active Hsp104 is toward the prion aggregates.
Hsp104 or its bacterial homolog, ClpB, are no prion specific chaperones, but act on different aggregated substrates to disaggregate them. For non-prion substrates, it is believed that Hsp100-type chaperones act in corporation with the Hsp70 chaperone machinery to disaggregate substrates.Citation115,Citation118-Citation120 For prion substrates however, the situation is less clear. It was demonstrated in vitro that Hsp104 by itself can server amyloid fibrils,Citation121,Citation122 but requires under certain experimental conditions additional factors,Citation123 and may act in concert with both Hsp40 and Hsp70 chaperones in vivo.Citation124,Citation125 Nevertheless, additional experimental evidence is required to answer this question. Regardless of whether Hsp40- and Hsp70 chaperones act in concert with Hsp104 or independently on prion aggregates, their crucial role in prion biogenesis is undoubed.Citation105-Citation108,Citation126,Citation127 Just to name very few examples, different [PSI+] variants can be partially cured by excess of the cytosolic Hsp70 member Ssa1/2 or a mutant thereof. Both situations also caused larger aggregates of fluorescent fusions of Sup35.Citation68,Citation75 Repressing the Hsp40-type chaperone Sis1 also leads to larger sizes of [PSI+] units and other prion aggregates.Citation125,Citation128
Furthermore, the actin cytoskeleton influences prion biogenesis, especially at early stages of prion formation. An early observation related to this is that the prion is partially curable by disrupting the actin cytoskeleton by the actin depolymerizing drug latrunculin A.Citation129 Moreover, proteins regulating the assembly and disassembly of actin filaments involved in formation of endocytic vesicles, such as for example Sla1, Sla2 and End3 interact with prion aggregates.Citation64-Citation66,Citation130 It was suggested that particularly during de novo formation of the [PSI+] prion, the misfolded prion determinants, eg Sup35 or NM-GFP, associate with the cytoskeleton, which may facilitate local concentration and subsequently conformational conversionCitation131 of the prion determinants to the prion state.Citation65,Citation86 A possible molecular link between the actin cytoskeleton and prion aggregates, especially during stress induced prion formation, is the stress-inducible protein Lsb2.Citation86 Lsb2 associates with actin patches, likely through it’s SH3 domain via Las17 and/or ubiquitin, and can bind to Sup35. A model was proposed where Lsb2 triggers accumulations of misfolded Sup35 at cortical actin cytoskeleton locations, especially during stress, and further events involving cytoskeletal networks would target the aggregates to perivacuolar quality control compartments such as for example the IPOD.Citation86 In line with this, actin has also been implicated in retention of other, non-amyloidogenic aggregated proteins in mother cells during cell division.Citation101,Citation102
Interfering with components of the ubiquitin-proteasome pathway was also identified to influence prion induction, mostly when triggered by overexpression of the prion determining region of [PSI+], but the effects may not be due to a direct interaction with Sup35, but feed into the pathway of stress induced prion formation.Citation14,Citation86,Citation110,Citation111
Model for Cellular Organization of Prion Aggregates During Induction and in the Mature Prion State
Different GFP-tagged versions of the Sup35 prion domain display different aggregation patterns when expressed at different levels (compare above and ), ranging from multiple aggregates to 1 single aggregate at the IPOD quality control compartment. These aggregates do very likely not represent the sole pool of heritable prion units that were referred to as propagons,Citation32,Citation132 simply because the propagon number with several hundredCitation133 seems much higher than the number of visible aggregates. This means that next to visible aggregates, smaller units of prion aggregates must exist. Indeed, diffusible units of NM-GFP of a size of 8–50 Sup35 moleculesCitation42 that are transferred to daughter cells during cell division, have been observed.Citation51 In a subsequent study, it was even proposed that these small units of prion aggregates could be fibrillar units.Citation57 It is still an open question whether there is an equilibrium between aggregates visible by fluorescence microscopy at the cell periphery and at quality control compartments such as the IPOD, but it seems possible that the smaller prion aggregate units visualized by fluorescence correlation spectroscopy (FCS)Citation51 could convert monomers and grow in size. The ratio between different types of aggregates may be controlled by different parameters known to be involved in prion biogenesis, such as for example the concentration of monomers, rate of prion fragmentation, which depends on the activity of Hsp104 toward prion fibrils, and biophysical properties of the fibrils such as frangibility.Citation31 This makes it tempting to speculate that very small aggregates below the size resolution of fluorescence microscopy, smaller visible aggregates and aggregates located at quality control compartments, could be in equilibrium with each others and led to the following model of how the different aggregation patterns may be involved in prion biology.
See . Prion induction is facilitated by accumulation of misfolded Sup35 at cortical actin structuresCitation65,Citation66,Citation86 and the IPOD compartment,Citation58 where the [PSI+] prion induction factor Rnq1 is present as well.Citation58,Citation95 The actin cytoskeleton and Lsb2 are involved in collecting misfolded Sup35 and depositing it at protein quality control compartments such as the IPOD, but maybe also at additional sites.Citation134 Additional proteins may be involved in this process as well.Citation109 Direct cross-seeding with pre-existing aggregates of [RNQ+] is likely.Citation93,Citation98,Citation99 Initial fiber seeds may grow rapidly by adding on monomers to the few open fiber ends. Hsp104 acts on prion fibrils in the deposits,Citation51,Citation58,Citation70,Citation95 but the limited number of fibrils and very high concentrations of monomers cause elongated fiber growth as net-behavior. Consequently, larger aggregates appear, which manifest themselves as the huge cytoplasmic rings when expression levels of Sup35 or NM-GFP are high.Citation46,Citation58,Citation65,Citation66 Hsp104 is likely to act on these larger aggregates to generate some smaller units nonetheless, as cells with ring aggregates can propagate this aggregation state, albeit less stable than a mature prion state. In addition, Hsp104 activity toward the fibers might be restricted by some unknown mechanism in these early induction states.Citation58
See . Eventually however, the number of fragments increases due to ongoing fragmentation by Hsp104, and monomers get added on more often, resulting in the reduction of their concentration. This situation causes slower fiber growth at constant fragmentation rates, which generates even shorter fragments. The more and shorter the prion aggregates become, the less likely their transmission is limited by a size threshold,Citation70,Citation73 which ensures inheritance of more prion units to progeny and a more stable inheritance. In this mature prion state, there is an equilibrium between small aggregates below the size resolution of fluorescence microscopy,Citation51,Citation57 larger peripheral aggregates and the deposits at the IPOD quality control compartment, which is controlled by the concentration of monomers, rate of fiber growth, rate of fiber cleavage and targeting of prion aggregates to the IPOD quality control compartment. It is currently unknown if and which additional factors control these sorting processes.
Closing Remarks
Recent efforts in studying prion aggregates have already revealed surprising connections between various different patterns of aggregation that were not foreseen initially when they were discovered. To further advance our understanding in the different aggregation patterns of prions and their relation with prion biogenesis, and to integrate them into cell physiology, it will be necessary to further unravel the complex interplay between cellular factors and prions. For example, we started to get some insights into the cellular principles of how cells can organize misfolded proteins in general, and amyloids and prions more specifically. However, another parameter, which is the turn-over of the aggregates and the fate of the amyloidogenic proteins they are composed of, is only poorly understood. Increasing knowledge about protein aggregate handling in the cell combined with advanced techniques of high-throughput-screening, modern fluorescence microscopy and electron microscopy should help to further unravel these unanswered questions in our understanding of yeast prion biogenesis.
Note Added in Proof. During the review process of this mini-review, Halfmann et al. (2012. Nature. doi: 10.1038/nature10875) published a paper about the occurrence of prions in wild yeast strains, an issue also discussed in this review. Halfmann et al. observed (PSI+) and (MOT3+) prions in many wild strains, where they conferred diverse phenotypes. Several of these were beneficial under selective pressure.
Acknowledgments
I would like to thank Dr. M. McCaffery for taking and kindly providing electron microscopy images, Dr. T. Serio for kindly providing fluorescence images of N-GFP-MC cells, Brooke Bevis and Susan Lindquist for kindly providing fluorescence images of NM-GFP at endogenous expression levels, Yves Cully for assistance with graphical elements in the figures, and Dr. A. Mogk for critical comments on the manuscript. The work was supported by a grant of the Deutsche Forschungsgemeinschaft to J.T. (TY93/1–1).
References
- Wickner RB. [URE3] as an altered URE2 protein: evidence for a prion analog in Saccharomyces cerevisiae. Science 1994; 264:566 - 9; http://dx.doi.org/10.1126/science.7909170; PMID: 7909170
- Prusiner SB. Novel proteinaceous infectious particles cause scrapie. Science 1982; 216:136 - 44; http://dx.doi.org/10.1126/science.6801762; PMID: 6801762
- Alberti S, Halfmann R, King O, Kapila A, Lindquist S. A systematic survey identifies prions and illuminates sequence features of prionogenic proteins. Cell 2009; 137:146 - 58; http://dx.doi.org/10.1016/j.cell.2009.02.044; PMID: 19345193
- Halfmann R, Alberti S, Lindquist S. Prions, protein homeostasis, and phenotypic diversity. Trends Cell Biol 2010; 20:125 - 33; http://dx.doi.org/10.1016/j.tcb.2009.12.003; PMID: 20071174
- Tuite MF, Serio TR. The prion hypothesis: from biological anomaly to basic regulatory mechanism. Nat Rev Mol Cell Biol 2010; 11:823 - 33; http://dx.doi.org/10.1038/nrm3007; PMID: 21081963
- Crow ET, Li L. Newly identified prions in budding yeast, and their possible functions. Semin Cell Dev Biol 2011; 22:452 - 9; http://dx.doi.org/10.1016/j.semcdb.2011.03.003; PMID: 21397710
- Nakayashiki T, Kurtzman CP, Edskes HK, Wickner RB. Yeast prions [URE3] and [PSI+] are diseases. Proc Natl Acad Sci U S A 2005; 102:10575 - 80; http://dx.doi.org/10.1073/pnas.0504882102; PMID: 16024723
- True HL, Lindquist SL. A yeast prion provides a mechanism for genetic variation and phenotypic diversity. Nature 2000; 407:477 - 83; http://dx.doi.org/10.1038/35035005; PMID: 11028992
- True HL, Berlin I, Lindquist SL. Epigenetic regulation of translation reveals hidden genetic variation to produce complex traits. Nature 2004; 431:184 - 7; http://dx.doi.org/10.1038/nature02885; PMID: 15311209
- Namy O, Galopier A, Martini C, Matsufuji S, Fabret C, Rousset JP. Epigenetic control of polyamines by the prion [PSI+]. [PSI+] Nat Cell Biol 2008; 10:1069 - 75; http://dx.doi.org/10.1038/ncb1766; PMID: 19160487
- Harrison LB, Yu Z, Stajich JE, Dietrich FS, Harrison PM. Evolution of budding yeast prion-determinant sequences across diverse fungi. J Mol Biol 2007; 368:273 - 82; http://dx.doi.org/10.1016/j.jmb.2007.01.070; PMID: 17320905
- Tuite MF, Mundy CR, Cox BS. Agents that cause a high frequency of genetic change from [psi+] to [psi-] in Saccharomyces cerevisiae. Genetics 1981; 98:691 - 711; PMID: 7037537
- Eaglestone SS, Cox BS, Tuite MF. Translation termination efficiency can be regulated in Saccharomyces cerevisiae by environmental stress through a prion-mediated mechanism. EMBO J 1999; 18:1974 - 81; http://dx.doi.org/10.1093/emboj/18.7.1974; PMID: 10202160
- Tyedmers J, Madariaga ML, Lindquist S. Prion switching in response to environmental stress. PLoS Biol 2008; 6:e294; http://dx.doi.org/10.1371/journal.pbio.0060294; PMID: 19067491
- Sideri TC, Stojanovski K, Tuite MF, Grant CM. Ribosome-associated peroxiredoxins suppress oxidative stress-induced de novo formation of the [PSI+] prion in yeast. Proc Natl Acad Sci U S A 2010; 107:6394 - 9; http://dx.doi.org/10.1073/pnas.1000347107; PMID: 20308573
- Masel J, Bergman A. The evolution of the evolvability properties of the yeast prion [PSI+]. [PSI+] Evolution 2003; 57:1498 - 512; PMID: 12940355
- Lancaster AK, Bardill JP, True HL, Masel J. The spontaneous appearance rate of the yeast prion [PSI+] and its implications for the evolution of the evolvability properties of the [PSI+] system. Genetics 2010; 184:393 - 400; http://dx.doi.org/10.1534/genetics.109.110213; PMID: 19917766
- Halfmann R, Lindquist S. Epigenetics in the extreme: prions and the inheritance of environmentally acquired traits. Science 2010; 330:629 - 32; http://dx.doi.org/10.1126/science.1191081; PMID: 21030648
- Derkatch IL, Liebman SW. Prion-prion interactions. Prion 2007; 1:161 - 9; http://dx.doi.org/10.4161/pri.1.3.4837; PMID: 19164893
- Tuite MF, Marchante R, Kushnirov V. Fungal prions: structure, function and propagation. Top Curr Chem 2011; 305:257 - 98; http://dx.doi.org/10.1007/128_2011_172; PMID: 21717344
- Derkatch IL, Chernoff YO, Kushnirov VV, Inge-Vechtomov SG, Liebman SW. Genesis and variability of [PSI] prion factors in Saccharomyces cerevisiae. Genetics 1996; 144:1375 - 86; PMID: 8978027
- Kochneva-Pervukhova NV, Chechenova MB, Valouev IA, Kushnirov VV, Smirnov VN, Ter-Avanesyan MD. [Psi(+)] prion generation in yeast: characterization of the ‘strain’ difference. Yeast 2001; 18:489 - 97; http://dx.doi.org/10.1002/yea.700; PMID: 11284005
- Schlumpberger M, Prusiner SB, Herskowitz I. Induction of distinct [URE3] yeast prion strains. Mol Cell Biol 2001; 21:7035 - 46; http://dx.doi.org/10.1128/MCB.21.20.7035-7046.2001; PMID: 11564886
- Bradley ME, Edskes HK, Hong JY, Wickner RB, Liebman SW. Interactions among prions and prion “strains” in yeast. Proc Natl Acad Sci U S A 2002; 99:Suppl 4 16392 - 9; http://dx.doi.org/10.1073/pnas.152330699; PMID: 12149514
- King CY, Diaz-Avalos R. Protein-only transmission of three yeast prion strains. Nature 2004; 428:319 - 23; http://dx.doi.org/10.1038/nature02391; PMID: 15029195
- Tanaka M, Chien P, Naber N, Cooke R, Weissman JS. Conformational variations in an infectious protein determine prion strain differences. Nature 2004; 428:323 - 8; http://dx.doi.org/10.1038/nature02392; PMID: 15029196
- Krishnan R, Lindquist SL. Structural insights into a yeast prion illuminate nucleation and strain diversity. Nature 2005; 435:765 - 72; http://dx.doi.org/10.1038/nature03679; PMID: 15944694
- Kalastavadi T, True HL. Analysis of the [RNQ+] prion reveals stability of amyloid fibers as the key determinant of yeast prion variant propagation. J Biol Chem 2010; 285:20748 - 55; http://dx.doi.org/10.1074/jbc.M110.115303; PMID: 20442412
- Uptain SM, Sawicki GJ, Caughey B, Lindquist S. Strains of [PSI(+)] are distinguished by their efficiencies of prion-mediated conformational conversion. EMBO J 2001; 20:6236 - 45; http://dx.doi.org/10.1093/emboj/20.22.6236; PMID: 11707395
- Diaz-Avalos R, King CY, Wall J, Simon M, Caspar DL. Strain-specific morphologies of yeast prion amyloid fibrils. Proc Natl Acad Sci U S A 2005; 102:10165 - 70; http://dx.doi.org/10.1073/pnas.0504599102; PMID: 16006506
- Tanaka M, Collins SR, Toyama BH, Weissman JS. The physical basis of how prion conformations determine strain phenotypes. Nature 2006; 442:585 - 9; http://dx.doi.org/10.1038/nature04922; PMID: 16810177
- Cox B, Ness F, Tuite M. Analysis of the generation and segregation of propagons: entities that propagate the [PSI+] prion in yeast. Genetics 2003; 165:23 - 33; PMID: 14504215
- Shorter J, Lindquist S. Prions as adaptive conduits of memory and inheritance. Nat Rev Genet 2005; 6:435 - 50; http://dx.doi.org/10.1038/nrg1616; PMID: 15931169
- Chernoff YO, Uptain SM, Lindquist SL. Analysis of prion factors in yeast. Methods Enzymol 2002; 351:499 - 538; http://dx.doi.org/10.1016/S0076-6879(02)51867-X; PMID: 12073366
- Tuite MF, Cox BS. Propagation of yeast prions. Nat Rev Mol Cell Biol 2003; 4:878 - 90; http://dx.doi.org/10.1038/nrm1247; PMID: 14625537
- Wickner RB, Edskes HK, Shewmaker F, Nakayashiki T. Prions of fungi: inherited structures and biological roles. Nat Rev Microbiol 2007; 5:611 - 8; http://dx.doi.org/10.1038/nrmicro1708; PMID: 17632572
- Alberti S, Halfmann R, Lindquist S. Biochemical, cell biological, and genetic assays to analyze amyloid and prion aggregation in yeast. Methods Enzymol 2010; 470:709 - 34; http://dx.doi.org/10.1016/S0076-6879(10)70030-6; PMID: 20946833
- Patino MM, Liu JJ, Glover JR, Lindquist S. Support for the prion hypothesis for inheritance of a phenotypic trait in yeast. Science 1996; 273:622 - 6; http://dx.doi.org/10.1126/science.273.5275.622; PMID: 8662547
- Paushkin SV, Kushnirov VV, Smirnov VN, Ter-Avanesyan MD. Propagation of the yeast prion-like [psi+] determinant is mediated by oligomerization of the SUP35-encoded polypeptide chain release factor. EMBO J 1996; 15:3127 - 34; PMID: 8670813
- Edskes HK, Gray VT, Wickner RB. The [URE3] prion is an aggregated form of Ure2p that can be cured by overexpression of Ure2p fragments. Proc Natl Acad Sci USA 1999; 96:1498 - 503; http://dx.doi.org/10.1073/pnas.96.4.1498; PMID: 9990052
- Sondheimer N, Lindquist S. Rnq1: an epigenetic modifier of protein function in yeast. Mol Cell 2000; 5:163 - 72; http://dx.doi.org/10.1016/S1097-2765(00)80412-8; PMID: 10678178
- Kryndushkin DS, Alexandrov IM, Ter-Avanesyan MD, Kushnirov VV. Yeast [PSI+] prion aggregates are formed by small Sup35 polymers fragmented by Hsp104. J Biol Chem 2003; 278:49636 - 43; http://dx.doi.org/10.1074/jbc.M307996200; PMID: 14507919
- Bagriantsev SN, Kushnirov VV, Liebman SW. Analysis of amyloid aggregates using agarose gel electrophoresis. Methods Enzymol 2006; 412:33 - 48; http://dx.doi.org/10.1016/S0076-6879(06)12003-0; PMID: 17046650
- Scherzinger E, Lurz R, Turmaine M, Mangiarini L, Hollenbach B, Hasenbank R, et al. Huntingtin-encoded polyglutamine expansions form amyloid-like protein aggregates in vitro and in vivo. Cell 1997; 90:549 - 58; http://dx.doi.org/10.1016/S0092-8674(00)80514-0; PMID: 9267034
- Wegrzyn RD, Bapat K, Newnam GP, Zink AD, Chernoff YO. Mechanism of prion loss after Hsp104 inactivation in yeast. Mol Cell Biol 2001; 21:4656 - 69; http://dx.doi.org/10.1128/MCB.21.14.4656-4669.2001; PMID: 11416143
- Zhou P, Derkatch IL, Liebman SW. The relationship between visible intracellular aggregates that appear after overexpression of Sup35 and the yeast prion-like elements [PSI(+)] and [PIN(+)]. Mol Microbiol 2001; 39:37 - 46; http://dx.doi.org/10.1046/j.1365-2958.2001.02224.x; PMID: 11123686
- Kimura Y, Koitabashi S, Fujita T. Analysis of yeast prion aggregates with amyloid-staining compound in vivo. Cell Struct Funct 2003; 28:187 - 93; http://dx.doi.org/10.1247/csf.28.187; PMID: 12951439
- Du Z, Park KW, Yu H, Fan Q, Li L. Newly identified prion linked to the chromatin-remodeling factor Swi1 in Saccharomyces cerevisiae. Nat Genet 2008; 40:460 - 5; http://dx.doi.org/10.1038/ng.112; PMID: 18362884
- Patel BK, Gavin-Smyth J, Liebman SW. The yeast global transcriptional co-repressor protein Cyc8 can propagate as a prion. Nat Cell Biol 2009; 11:344 - 9; http://dx.doi.org/10.1038/ncb1843; PMID: 19219034
- Rogoza T, Goginashvili A, Rodionova S, Ivanov M, Viktorovskaya O, Rubel A, et al. Non-Mendelian determinant [ISP+] in yeast is a nuclear-residing prion form of the global transcriptional regulator Sfp1. Proc Natl Acad Sci U S A 2010; 107:10573 - 7; http://dx.doi.org/10.1073/pnas.1005949107; PMID: 20498075
- Kawai-Noma S, Ayano S, Pack CG, Kinjo M, Yoshida M, Yasuda K, et al. Dynamics of yeast prion aggregates in single living cells. Genes Cells 2006; 11:1085 - 96; http://dx.doi.org/10.1111/j.1365-2443.2006.01004.x; PMID: 16923127
- Glover JR, Kowal AS, Schirmer EC, Patino MM, Liu JJ, Lindquist S. Self-seeded fibers formed by Sup35, the protein determinant of [PSI+], a heritable prion-like factor of S. cerevisiae. Cell 1997; 89:811 - 9; http://dx.doi.org/10.1016/S0092-8674(00)80264-0; PMID: 9182769
- Taylor KL, Cheng N, Williams RW, Steven AC, Wickner RB. Prion domain initiation of amyloid formation in vitro from native Ure2p. Science 1999; 283:1339 - 43; http://dx.doi.org/10.1126/science.283.5406.1339; PMID: 10037606
- Vitrenko YA, Gracheva EO, Richmond JE, Liebman SW. Visualization of aggregation of the Rnq1 prion domain and cross-seeding interactions with Sup35NM. J Biol Chem 2007; 282:1779 - 87; http://dx.doi.org/10.1074/jbc.M609269200; PMID: 17121829
- King CY, Tittmann P, Gross H, Gebert R, Aebi M, Wüthrich K. Prion-inducing domain 2-114 of yeast Sup35 protein transforms in vitro into amyloid-like filaments. Proc Natl Acad Sci USA 1997; 94:6618 - 22; http://dx.doi.org/10.1073/pnas.94.13.6618; PMID: 9192614
- Speransky VV, Taylor KL, Edskes HK, Wickner RB, Steven AC. Prion filament networks in [URE3] cells of Saccharomyces cerevisiae. J Cell Biol 2001; 153:1327 - 36; http://dx.doi.org/10.1083/jcb.153.6.1327; PMID: 11402074
- Kawai-Noma S, Pack CG, Kojidani T, Asakawa H, Hiraoka Y, Kinjo M, et al. In vivo evidence for the fibrillar structures of Sup35 prions in yeast cells. J Cell Biol 2010; 190:223 - 31; http://dx.doi.org/10.1083/jcb.201002149; PMID: 20643880
- Tyedmers J, Treusch S, Dong J, McCaffery JM, Bevis B, Lindquist S. Prion induction involves an ancient system for the sequestration of aggregated proteins and heritable changes in prion fragmentation. Proc Natl Acad Sci USA 2010; 107:8633 - 8; http://dx.doi.org/10.1073/pnas.1003895107; PMID: 20421488
- Krzewska J, Melki R. Molecular chaperones and the assembly of the prion Sup35p, an in vitro study. EMBO J 2006; 25:822 - 33; http://dx.doi.org/10.1038/sj.emboj.7600985; PMID: 16467849
- Baxa U, Keller PW, Cheng N, Wall JS, Steven AC. In Sup35p filaments (the [PSI+] prion), the globular C-terminal domains are widely offset from the amyloid fibril backbone. Mol Microbiol 2011; 79:523 - 32; http://dx.doi.org/10.1111/j.1365-2958.2010.07466.x; PMID: 21219467
- Brachmann A, Baxa U, Wickner RB. Prion generation in vitro: amyloid of Ure2p is infectious. EMBO J 2005; 24:3082 - 92; http://dx.doi.org/10.1038/sj.emboj.7600772; PMID: 16096644
- Patel BK, Liebman SW. “Prion-proof” for [PIN+]: infection with in vitro-made amyloid aggregates of Rnq1p-(132-405) induces [PIN+]. [PIN+] J Mol Biol 2007; 365:773 - 82; http://dx.doi.org/10.1016/j.jmb.2006.10.069; PMID: 17097676
- Du Z, Crow ET, Kang HS, Li L. Distinct subregions of Swi1 manifest striking differences in prion transmission and SWI/SNF function. Mol Cell Biol 2010; 30:4644 - 55; http://dx.doi.org/10.1128/MCB.00225-10; PMID: 20679490
- Bagriantsev SN, Gracheva EO, Richmond JE, Liebman SW. Variant-specific [PSI+] infection is transmitted by Sup35 polymers within [PSI+] aggregates with heterogeneous protein composition. Mol Biol Cell 2008; 19:2433 - 43; http://dx.doi.org/10.1091/mbc.E08-01-0078; PMID: 18353968
- Ganusova EE, Ozolins LN, Bhagat S, Newnam GP, Wegrzyn RD, Sherman MY, et al. Modulation of prion formation, aggregation, and toxicity by the actin cytoskeleton in yeast. Mol Cell Biol 2006; 26:617 - 29; http://dx.doi.org/10.1128/MCB.26.2.617-629.2006; PMID: 16382152
- Mathur V, Taneja V, Sun Y, Liebman SW. Analyzing the birth and propagation of two distinct prions, [PSI+] and [Het-s](y), in yeast. Mol Biol Cell 2010; 21:1449 - 61; http://dx.doi.org/10.1091/mbc.E09-11-0927; PMID: 20219972
- Satpute-Krishnan P, Serio TR. Prion protein remodelling confers an immediate phenotypic switch. Nature 2005; 437:262 - 5; http://dx.doi.org/10.1038/nature03981; PMID: 16148935
- Song Y, Wu YX, Jung G, Tutar Y, Eisenberg E, Greene LE, et al. Role for Hsp70 chaperone in Saccharomyces cerevisiae prion seed replication. Eukaryot Cell 2005; 4:289 - 97; http://dx.doi.org/10.1128/EC.4.2.289-297.2005; PMID: 15701791
- Wu YX, Greene LE, Masison DC, Eisenberg E. Curing of yeast [PSI+] prion by guanidine inactivation of Hsp104 does not require cell division. Proc Natl Acad Sci USA 2005; 102:12789 - 94; http://dx.doi.org/10.1073/pnas.0506384102; PMID: 16123122
- Satpute-Krishnan P, Langseth SX, Serio TR. Hsp104-dependent remodeling of prion complexes mediates protein-only inheritance. PLoS Biol 2007; 5:e24; http://dx.doi.org/10.1371/journal.pbio.0050024; PMID: 17253904
- Greene LE, Park YN, Masison DC, Eisenberg E. Application of GFP-labeling to study prions in yeast. Protein Pept Lett 2009; 16:635 - 41; http://dx.doi.org/10.2174/092986609788490221; PMID: 19519522
- Borchsenius AS, Müller S, Newnam GP, Inge-Vechtomov SG, Chernoff YO. Prion variant maintained only at high levels of the Hsp104 disaggregase. Curr Genet 2006; 49:21 - 9; http://dx.doi.org/10.1007/s00294-005-0035-0; PMID: 16307272
- Derdowski A, Sindi SS, Klaips CL, DiSalvo S, Serio TR. A size threshold limits prion transmission and establishes phenotypic diversity. Science 2010; 330:680 - 3; http://dx.doi.org/10.1126/science.1197785; PMID: 21030659
- Ness F, Ferreira P, Cox BS, Tuite MF. Guanidine hydrochloride inhibits the generation of prion “seeds” but not prion protein aggregation in yeast. Mol Cell Biol 2002; 22:5593 - 605; http://dx.doi.org/10.1128/MCB.22.15.5593-5605.2002; PMID: 12101251
- Mathur V, Hong JY, Liebman SW. Ssa1 overexpression and [PIN(+)] variants cure [PSI(+)] by dilution of aggregates. J Mol Biol 2009; 390:155 - 67; http://dx.doi.org/10.1016/j.jmb.2009.04.063; PMID: 19422835
- Borchsenius AS, Wegrzyn RD, Newnam GP, Inge-Vechtomov SG, Chernoff YO. Yeast prion protein derivative defective in aggregate shearing and production of new ‘seeds’. EMBO J 2001; 20:6683 - 91; http://dx.doi.org/10.1093/emboj/20.23.6683; PMID: 11726504
- Kawai-Noma S, Pack CG, Tsuji T, Kinjo M, Taguchi H. Single mother-daughter pair analysis to clarify the diffusion properties of yeast prion Sup35 in guanidine-HCl-treated [PSI] cells. Genes Cells 2009; 14:1045 - 54; http://dx.doi.org/10.1111/j.1365-2443.2009.01333.x; PMID: 19674118
- Romanova NV, Chernoff YO. Hsp104 and prion propagation. Protein Pept Lett 2009; 16:598 - 605; http://dx.doi.org/10.2174/092986609788490078; PMID: 19519517
- Newnam GP, Birchmore JL, Chernoff YO. Destabilization and recovery of a yeast prion after mild heat shock. J Mol Biol 2011; 408:432 - 48; http://dx.doi.org/10.1016/j.jmb.2011.02.034; PMID: 21392508
- Bradley ME, Liebman SW. Destabilizing interactions among [PSI(+)] and [PIN(+)] yeast prion variants. Genetics 2003; 165:1675 - 85; PMID: 14704158
- Lund PM, Cox BS. Reversion analysis of [psi-] mutations in Saccharomyces cerevisiae. Genet Res 1981; 37:173 - 82; http://dx.doi.org/10.1017/S0016672300020140; PMID: 7021322
- Liu JJ, Lindquist S. Oligopeptide-repeat expansions modulate ‘protein-only’ inheritance in yeast. Nature 1999; 400:573 - 6; http://dx.doi.org/10.1038/22919; PMID: 10448860
- Chernoff YO, Derkach IL, Inge-Vechtomov SG. Multicopy SUP35 gene induces de-novo appearance of psi-like factors in the yeast Saccharomyces cerevisiae. Curr Genet 1993; 24:268 - 70; http://dx.doi.org/10.1007/BF00351802; PMID: 8221937
- Ter-Avanesyan MD, Kushnirov VV, Dagkesamanskaya AR, Didichenko SA, Chernoff YO, Inge-Vechtomov SG, et al. Deletion analysis of the SUP35 gene of the yeast Saccharomyces cerevisiae reveals two non-overlapping functional regions in the encoded protein. Mol Microbiol 1993; 7:683 - 92; http://dx.doi.org/10.1111/j.1365-2958.1993.tb01159.x; PMID: 8469113
- Derkatch IL, Bradley ME, Zhou P, Chernoff YO, Liebman SW. Genetic and environmental factors affecting the de novo appearance of the [PSI+] prion in Saccharomyces cerevisiae. Genetics 1997; 147:507 - 19; PMID: 9335589
- Chernova TA, Romanyuk AV, Karpova TS, Shanks JR, Ali M, Moffatt N, et al. Prion induction by the short-lived, stress-induced protein Lsb2 is regulated by ubiquitination and association with the actin cytoskeleton. Mol Cell 2011; 43:242 - 52; http://dx.doi.org/10.1016/j.molcel.2011.07.001; PMID: 21777813
- Chernoff YO, Inge-Vechtomov SG, Derkach IL, Ptyushkina MV, Tarunina OV, Dagkesamanskaya AR, et al. Dosage-dependent translational suppression in yeast Saccharomyces cerevisiae. Yeast 1992; 8:489 - 99; http://dx.doi.org/10.1002/yea.320080702; PMID: 1523883
- Vishveshwara N, Bradley ME, Liebman SW. Sequestration of essential proteins causes prion associated toxicity in yeast. Mol Microbiol 2009; 73:1101 - 14; http://dx.doi.org/10.1111/j.1365-2958.2009.06836.x; PMID: 19682262
- Derkatch IL, Bradley ME, Masse SV, Zadorsky SP, Polozkov GV, Inge-Vechtomov SG, et al. Dependence and independence of [PSI(+)] and [PIN(+)]: a two-prion system in yeast?. EMBO J 2000; 19:1942 - 52; http://dx.doi.org/10.1093/emboj/19.9.1942; PMID: 10790361
- Fernandez-Bellot E, Guillemet E, Cullin C. The yeast prion [URE3] can be greatly induced by a functional mutated URE2 allele. EMBO J 2000; 19:3215 - 22; http://dx.doi.org/10.1093/emboj/19.13.3215; PMID: 10880435
- Derkatch IL, Bradley ME, Hong JY, Liebman SW. Prions affect the appearance of other prions: the story of [PIN(+)]. Cell 2001; 106:171 - 82; http://dx.doi.org/10.1016/S0092-8674(01)00427-5; PMID: 11511345
- Osherovich LZ, Weissman JS. Multiple Gln/Asn-rich prion domains confer susceptibility to induction of the yeast [PSI(+)] prion. Cell 2001; 106:183 - 94; http://dx.doi.org/10.1016/S0092-8674(01)00440-8; PMID: 11511346
- Salnikova AB, Kryndushkin DS, Smirnov VN, Kushnirov VV, Ter-Avanesyan MD. Nonsense suppression in yeast cells overproducing Sup35 (eRF3) is caused by its non-heritable amyloids. J Biol Chem 2005; 280:8808 - 12; http://dx.doi.org/10.1074/jbc.M410150200; PMID: 15618222
- Taneja V, Maddelein ML, Talarek N, Saupe SJ, Liebman SW. A non-Q/N-rich prion domain of a foreign prion, [Het-s], can propagate as a prion in yeast. Mol Cell 2007; 27:67 - 77; http://dx.doi.org/10.1016/j.molcel.2007.05.027; PMID: 17612491
- Kaganovich D, Kopito R, Frydman J. Misfolded proteins partition between two distinct quality control compartments. Nature 2008; 454:1088 - 95; http://dx.doi.org/10.1038/nature07195; PMID: 18756251
- He C, Klionsky DJ. Regulation mechanisms and signaling pathways of autophagy. Annu Rev Genet 2009; 43:67 - 93; http://dx.doi.org/10.1146/annurev-genet-102808-114910; PMID: 19653858
- Nakatogawa H, Suzuki K, Kamada Y, Ohsumi Y. Dynamics and diversity in autophagy mechanisms: lessons from yeast. Nat Rev Mol Cell Biol 2009; 10:458 - 67; http://dx.doi.org/10.1038/nrm2708; PMID: 19491929
- Derkatch IL, Uptain SM, Outeiro TF, Krishnan R, Lindquist SL, Liebman SW. Effects of Q/N-rich, polyQ, and non-polyQ amyloids on the de novo formation of the [PSI+] prion in yeast and aggregation of Sup35 in vitro. Proc Natl Acad Sci U S A 2004; 101:12934 - 9; http://dx.doi.org/10.1073/pnas.0404968101; PMID: 15326312
- Kimura Y, Koitabashi S, Kakizuka A, Fujita T. The role of pre-existing aggregates in Hsp104-dependent polyglutamine aggregate formation and epigenetic change of yeast prions. Genes Cells 2004; 9:685 - 96; http://dx.doi.org/10.1111/j.1356-9597.2004.00759.x; PMID: 15298677
- Tyedmers J, Mogk A, Bukau B. Cellular strategies for controlling protein aggregation. Nat Rev Mol Cell Biol 2010; 11:777 - 88; http://dx.doi.org/10.1038/nrm2993; PMID: 20944667
- Aguilaniu H, Gustafsson L, Rigoulet M, Nyström T. Asymmetric inheritance of oxidatively damaged proteins during cytokinesis. Science 2003; 299:1751 - 3; http://dx.doi.org/10.1126/science.1080418; PMID: 12610228
- Liu B, Larsson L, Caballero A, Hao X, Oling D, Grantham J, et al. The polarisome is required for segregation and retrograde transport of protein aggregates. Cell 2010; 140:257 - 67; http://dx.doi.org/10.1016/j.cell.2009.12.031; PMID: 20141839
- Winkler J, Seybert A, König L, Pruggnaller S, Haselmann U, Sourjik V, et al. Quantitative and spatio-temporal features of protein aggregation in Escherichia coli and consequences on protein quality control and cellular ageing. EMBO J 2010; 29:910 - 23; http://dx.doi.org/10.1038/emboj.2009.412; PMID: 20094032
- Tessarz P, Schwarz M, Mogk A, Bukau B. The yeast AAA+ chaperone Hsp104 is part of a network that links the actin cytoskeleton with the inheritance of damaged proteins. Mol Cell Biol 2009; 29:3738 - 45; http://dx.doi.org/10.1128/MCB.00201-09; PMID: 19398583
- Chernoff YO. Stress and prions: lessons from the yeast model. FEBS Lett 2007; 581:3695 - 701; http://dx.doi.org/10.1016/j.febslet.2007.04.075; PMID: 17509571
- Perrett S, Jones GW. Insights into the mechanism of prion propagation. Curr Opin Struct Biol 2008; 18:52 - 9; http://dx.doi.org/10.1016/j.sbi.2007.12.005; PMID: 18243685
- Tuite M, Stojanovski K, Ness F, Merritt G, Koloteva-Levine N. Cellular factors important for the de novo formation of yeast prions. Biochem Soc Trans 2008; 36:1083 - 7; http://dx.doi.org/10.1042/BST0361083; PMID: 18793193
- Jones GW, Tuite MF. Chaperoning prions: the cellular machinery for propagating an infectious protein?. Bioessays 2005; 27:823 - 32; http://dx.doi.org/10.1002/bies.20267; PMID: 16015602
- Manogaran AL, Hong JY, Hufana J, Tyedmers J, Lindquist S, Liebman SW. Prion formation and polyglutamine aggregation are controlled by two classes of genes. PLoS Genet 2011; 7:e1001386; http://dx.doi.org/10.1371/journal.pgen.1001386; PMID: 21625618
- Chernova TA, Allen KD, Wesoloski LM, Shanks JR, Chernoff YO, Wilkinson KD. Pleiotropic effects of Ubp6 loss on drug sensitivities and yeast prion are due to depletion of the free ubiquitin pool. J Biol Chem 2003; 278:52102 - 15; http://dx.doi.org/10.1074/jbc.M310283200; PMID: 14559899
- Allen KD, Chernova TA, Tennant EP, Wilkinson KD, Chernoff YO. Effects of ubiquitin system alterations on the formation and loss of a yeast prion. J Biol Chem 2007; 282:3004 - 13; http://dx.doi.org/10.1074/jbc.M609597200; PMID: 17142456
- Redeker V, Hughes C, Savistchenko J, Vissers JP, Melki R. Qualitative and quantitative multiplexed proteomic analysis of complex yeast protein fractions that modulate the assembly of the yeast prion Sup35p. PLoS One 2011; 6:e23659; http://dx.doi.org/10.1371/journal.pone.0023659; PMID: 21931608
- Chernoff YO, Lindquist SL, Ono B, Inge-Vechtomov SG, Liebman SW. Role of the chaperone protein Hsp104 in propagation of the yeast prion-like factor [psi+]. [psi+] Science 1995; 268:880 - 4; http://dx.doi.org/10.1126/science.7754373; PMID: 7754373
- Sweeny EA, Shorter J. Prion proteostasis: Hsp104 meets its supporting cast. Prion 2008; 2:135 - 40; http://dx.doi.org/10.4161/pri.2.4.7952; PMID: 19242125
- Haslberger T, Bukau B, Mogk A. Towards a unifying mechanism for ClpB/Hsp104-mediated protein disaggregation and prion propagation. Biochem Cell Biol 2010; 88:63 - 75; http://dx.doi.org/10.1139/O09-118; PMID: 20130680
- Ferreira PC, Ness F, Edwards SR, Cox BS, Tuite MF. The elimination of the yeast [PSI+] prion by guanidine hydrochloride is the result of Hsp104 inactivation. Mol Microbiol 2001; 40:1357 - 69; http://dx.doi.org/10.1046/j.1365-2958.2001.02478.x; PMID: 11442834
- Jung G, Masison DC. Guanidine hydrochloride inhibits Hsp104 activity in vivo: a possible explanation for its effect in curing yeast prions. Curr Microbiol 2001; 43:7 - 10; http://dx.doi.org/10.1007/s002840010251; PMID: 11375656
- Glover JR, Lindquist S. Hsp104, Hsp70, and Hsp40: a novel chaperone system that rescues previously aggregated proteins. Cell 1998; 94:73 - 82; http://dx.doi.org/10.1016/S0092-8674(00)81223-4; PMID: 9674429
- Weibezahn J, Tessarz P, Schlieker C, Zahn R, Maglica Z, Lee S, et al. Thermotolerance requires refolding of aggregated proteins by substrate translocation through the central pore of ClpB. Cell 2004; 119:653 - 65; http://dx.doi.org/10.1016/j.cell.2004.11.027; PMID: 15550247
- Zietkiewicz S, Krzewska J, Liberek K. Successive and synergistic action of the Hsp70 and Hsp100 chaperones in protein disaggregation. J Biol Chem 2004; 279:44376 - 83; http://dx.doi.org/10.1074/jbc.M402405200; PMID: 15302880
- Shorter J, Lindquist S. Hsp104 catalyzes formation and elimination of self-replicating Sup35 prion conformers. Science 2004; 304:1793 - 7; http://dx.doi.org/10.1126/science.1098007; PMID: 15155912
- Shorter J, Lindquist S. Destruction or potentiation of different prions catalyzed by similar Hsp104 remodeling activities. Mol Cell 2006; 23:425 - 38; http://dx.doi.org/10.1016/j.molcel.2006.05.042; PMID: 16885031
- Inoue Y, Taguchi H, Kishimoto A, Yoshida M. Hsp104 binds to yeast Sup35 prion fiber but needs other factor(s) to sever it. J Biol Chem 2004; 279:52319 - 23; http://dx.doi.org/10.1074/jbc.M408159200; PMID: 15448141
- Tipton KA, Verges KJ, Weissman JS. In vivo monitoring of the prion replication cycle reveals a critical role for Sis1 in delivering substrates to Hsp104. Mol Cell 2008; 32:584 - 91; http://dx.doi.org/10.1016/j.molcel.2008.11.003; PMID: 19026788
- Higurashi T, Hines JK, Sahi C, Aron R, Craig EA. Specificity of the J-protein Sis1 in the propagation of 3 yeast prions. Proc Natl Acad Sci U S A 2008; 105:16596 - 601; http://dx.doi.org/10.1073/pnas.0808934105; PMID: 18955697
- Masison DC, Kirkland PA, Sharma D. Influence of Hsp70s and their regulators on yeast prion propagation. Prion 2009; 3:65 - 73; http://dx.doi.org/10.4161/pri.3.2.9134; PMID: 19556854
- Summers DW, Douglas PM, Cyr DM. Prion propagation by Hsp40 molecular chaperones. Prion 2009; 3:59 - 64; http://dx.doi.org/10.4161/pri.3.2.9062; PMID: 19535913
- Aron R, Higurashi T, Sahi C, Craig EA. J-protein co-chaperone Sis1 required for generation of [RNQ+] seeds necessary for prion propagation. EMBO J 2007; 26:3794 - 803; http://dx.doi.org/10.1038/sj.emboj.7601811; PMID: 17673909
- Bailleul-Winslett PA, Newnam GP, Wegrzyn RD, Chernoff YO. An antiprion effect of the anticytoskeletal drug latrunculin A in yeast. Gene Expr 2000; 9:145 - 56; PMID: 11243411
- Bailleul PA, Newnam GP, Steenbergen JN, Chernoff YO. Genetic study of interactions between the cytoskeletal assembly protein sla1 and prion-forming domain of the release factor Sup35 (eRF3) in Saccharomyces cerevisiae. Genetics 1999; 153:81 - 94; PMID: 10471702
- Serio TR, Cashikar AG, Kowal AS, Sawicki GJ, Moslehi JJ, Serpell L, et al. Nucleated conformational conversion and the replication of conformational information by a prion determinant. Science 2000; 289:1317 - 21; http://dx.doi.org/10.1126/science.289.5483.1317; PMID: 10958771
- Eaglestone SS, Ruddock LW, Cox BS, Tuite MF. Guanidine hydrochloride blocks a critical step in the propagation of the prion-like determinant [PSI(+)] of Saccharomyces cerevisiae. Proc Natl Acad Sci U S A 2000; 97:240 - 4; http://dx.doi.org/10.1073/pnas.97.1.240; PMID: 10618402
- Byrne LJ, Cole DJ, Cox BS, Ridout MS, Morgan BJ, Tuite MF. The number and transmission of [PSI] prion seeds (Propagons) in the yeast Saccharomyces cerevisiae. PLoS One 2009; 4:e4670; http://dx.doi.org/10.1371/journal.pone.0004670; PMID: 19262693
- Wang Y, Meriin AB, Zaarur N, Romanova NV, Chernoff YO, Costello CE, et al. Abnormal proteins can form aggresome in yeast: aggresome-targeting signals and components of the machinery. FASEB J 2009; 23:451 - 63; http://dx.doi.org/10.1096/fj.08-117614; PMID: 18854435