Abstract
Biomaterials, having evolved over millions of years, often exceed man‑made materials in their properties. Spider silk is one outstanding fibrous biomaterial which consists almost entirely of large proteins. Silk fibers have tensile strengths comparable to steel and some silks are nearly as elastic as rubber on a weight to weight basis. In combining these two properties, silks reveal a toughness that is two to three times that of synthetic fibers like Nylon or Kevlar. Spider silk is also antimicrobial, hypoallergenic and completely biodegradable.
This article focuses on the structure‑function relationship of the characterized highly repetitive spider silk spidroins and their conformational conversion from solution into fibers. Such knowedge is of crucial importance to understanding the intrinsic properties of spider silk and to get insight into the sophisticated assembly processes of silk proteins. This review further outlines recent progress in recombinant production of spider silk proteins and their assembly into distinct polymer materials as a basis for novel products.
Acknowledgements
We thank John Hardy and Eileen Lintz for critical comments on the manuscript. This work was supported by the DFG (SCHE 603/4-3).
This manuscript has been previously published: Römer L. Scheibel T. The elaborate structure of spider silk; Structure and function of a natural high performance fiber. Fibrous, Proteins Scheibel T. Austin Landes Bioscience 2008; 121 - 129
Figures and Tables
Figure 1 (A) Schematic overview of different types of spider silk as produced by female orb weaving spiders such as the European garden spider Araneus diadematus. (B) Electronmicrographs of natural spider silk taken from an orb web of Araneus diadematus. The various morphologies of the distinct silk types can be easily depicted. The inset shows the smooth surface of MA silk.
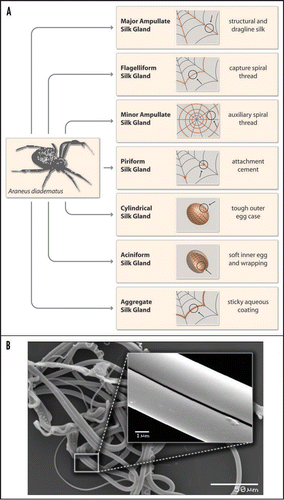
Figure 2 (A) Amino acid composition of three common proteins in comparison to spider silk. (B) Model of the hierarchical structure of a MA silk protein. For example, Araneus diadematus Fibroin-3 (ADF3) has a highly repetitive core domain flanked by two nonrepetitive domains (aminoterminal domain: NRN; carboxyterminal domain: NRc). The aminoterminal domain also comprises a secretion signal sequence to allow protein export. (C) Amino acid motifs of silk proteins. The different amino acid motifs present in MA and Flag silk are correlated with their putative structure and their impact on the final properties of the thread. Although the function of the nonrepetitive parts is not fully understood, it is thought that these parts play a role in triggering the assembly of spider silks.
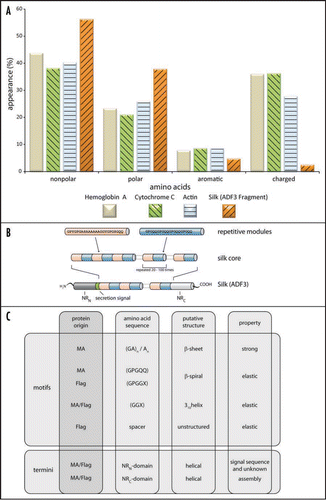
Figure 3 Schematic structure of spider MA silk. The thread is composed of small crystalline b-sheet rich subunits (see close-ups) which are embedded into an amorphous structure. The crystalline and noncrystalline parts are covalently connected, ensuring the coexistence of strength and ductility. Diameters of MA threads depend on species, but also on age, weight and state of health of a specific individual.
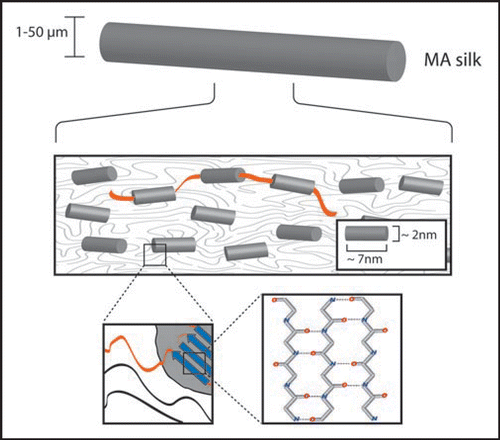
Figure 4 Model of the silk spinning process. The highly concentrated spider silk protein solution is secreted and stored inside the spinning gland. Upon initiation of thread formation, the solution is directed through a narrow ion exchange channel, in which a phase separation process takes place. Mechanical drawing of the silk thread (hind legs/gravity) results in rapid assembly of the silk fiber. Two theories exist concerning the molecular process inside the spinning duct. The first theory supports a liquid-crystalline behavior of the silk proteins, whereas the other theory favors a micellar organization of the proteins, before elongation (through laminar force) finally leads to thread assembly.
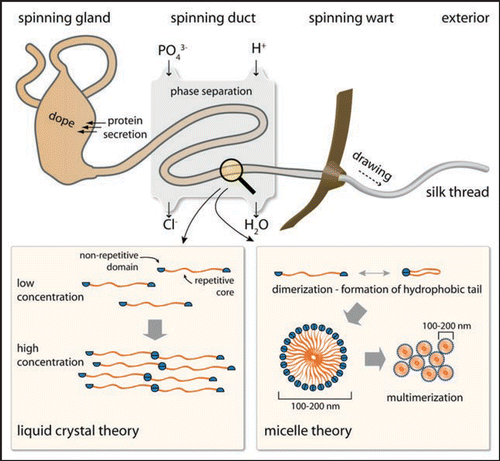
Figure 5 Possible shapes of spider silk. In nature, spider silk proteins are exclusively converted into silk threads. However, in vitro it is possible to transform silk proteins in other two- or three-dimensional shapes. The figure shows images of a capsule, a sphere, a thread and nano-fibrils (all electron microscopy), as well as a hydrogel and a film (photograph) made by recombinantly produced engineered spider silk protein.Citation35,Citation42,Citation48,Citation71
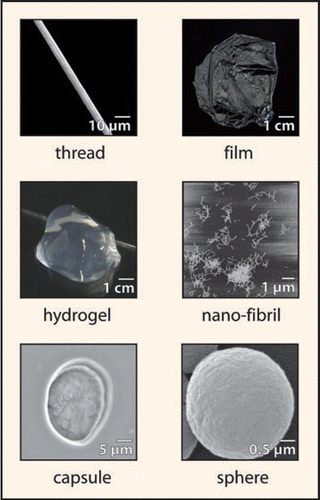
Table 1 Mechanical properties of natural and synthetic fibers (taken from refs. Citation34 and Citation47)