Abstract
Transmissible spongiform encephalopathies (prion diseases) in animals may be associated with a zoonotic risk potential for humans as shown by the occurrence of variant Creutzfeldt-Jakob disease in the wake of the bovine spongiform encephalopathy epidemic. Thus, the increasing exposure of humans in North America to cervid prions of chronic wasting disease (CWD) in elk and deer has prompted comprehensive risk assessments. The susceptibility of humans to CWD infections is currently under investigation in different studies using macaques as primate models. The necessity for such studies was recently reinforced when disease-associated prion protein and its seeding activity were detected in muscles of clinically inconspicuous CWD-infected white-tailed deer (WTD). Increasing evidence points to the existence of different CWD strains, and CWD prions may also change or newly emerge over time. Therefore, CWD isolates examined in macaques should be characterized as precisely as possible for their molecular identity. On this basis other CWD field samples collected in the past, present or future could be systematically compared with macaque-tested inocula in order to assess whether they are covered by the ongoing risk assessments in primates. CWD typing by Fourier transform-infrared spectroscopy of pathological prion protein may provide a method of choice for this purpose.
Transmissible Spongiform Encephalopathies: Prion Diseases with a Zoonotic Risk Potential
Transmissible spongiform encephalopathies (TSEs) are fatal neurodegenerative diseases caused by prions (proteinaceous infectious particles) such as chronic wasting disease (CWD) in cervids, scrapie in sheep, bovine spongiform encephalopathy (BSE) or human Creutzfeldt-Jakob disease (CJD).Citation1 While some TSEs have been known long (e.g., scrapie or CJD), others emerged only in recent years. Of the latter, BSE and variant Creutzfeldt-Jakob disease (vCJD) attracted great attention in science, politics and the media. The occurrence of vCJD in the wake of the BSE epidemic showed that animal prions may potentially breach the species barrier to humans via the alimentary route.Citation2 This hazard has called for a stringent surveillance and control of prion reservoirs in farmed livestock and hunted game.
CWD and other prions are thought to consist essentially of host-encoded prion protein (PrP) with a pathological, β-sheet rich folding- and aggregation structure, referred to as PrPSc or PrPTSE.Citation1,Citation3,Citation4 Their replication is putatively mediated by a process of seeded polymerization in which seeding-active nuclei of PrPTSE recruit cellular prion protein (PrPC) and incorporate it, in a misfolded form, into growing amyloid-like PrP aggregates. When such polymers break up into smaller units this leads to a multiplication of PrP particles with proteinaceous seeding activity further mediating the autocatalytic replication of the pathological protein state. Prion-associated seeding activity converting normal protease-sensitive PrPC into Proteinase K-resistant prion protein (PrPres) can be monitored in vitro by protein misfolding cyclic amplification (PMCA).Citation5
Exposure of Humans to CWD Prions
Chronic wasting disease is a TSE in white-tailed deer (WTD), mule deer, Rocky Mountain elk and moose. Over the past years this disease has shown a sustained spread in captive and free-ranging cervids in North America.Citation6,Citation7 The increasingly frequent and widespread occurrence of affected animals is likely to augment the exposure of humans to the CWD agent. Prion infectivity or TSE-associated prion protein have been detected in the central and peripheral nervous system, in a variety of lymphoid tissues as well as in heart muscle, blood, saliva, feces and urine of CWD-infected cervids.Citation7 Also, infectious CWD agent was found in antler velvet of elk and in skeletal muscles of mule deer with CWD.Citation8,Citation9 Thus, people processing cervid carcasses, users of medicinal products made from antler velvet and venison consumers may be exposed to an elevated risk for contamination with CWD prions.
Recently, PrPTSE and its proteinaceous seeding activity could be directly demonstrated for the first time in skeletal muscles of CWD-infected cervids.Citation10 The animals examined in this study were farmed and free-ranging WTD for which no clinical signs of CWD had been recognized. However, they had been officially confirmed positive for CWD based on the detection of PrPTSE in brain tissue or lymph nodes and were thus apparently in a state of pre- or subclinical infection. Muscles from such clinically inconspicuous carrier animals appear more likely to enter the human food chain than meat from cervids that show symptoms of CWD. Whether this may provide a relevant mode for the inadvertent foodborne transmission of CWD prions is still unclear. Yet, the presence and seeding activity of PrPTSE in skeletal muscles of pre- or subclinically infected WTD reinforced the need to comprehensively assess whether humans are susceptible to zoonotic CWD infections.
CWD Risk Assessments
Transmissibility to other animal species.
CWD has been observed under natural conditions so far only in cervids. However, the disease could be transmitted experimentally to recipients such as vole species, golden hamsters, minks, ferrets, goats and cattle.Citation7 Thus, in artificial setups CWD prions were able to breach the species barrier to different kinds of wild and domestic animals. It remains unknown, though, whether inter-species transmission of CWD would be also possible via natural routes of exposure such as contact to infected cervids or environments contaminated with CWD prions.
Transmissibility to humans.
The current state of epidemiological research suggests a rather robust barrier for the transmission of CWD to humans. Particularly, the surveillance of human prion diseases in areas with a long history of endemic CWD such as Colorado and Wyoming did not reveal evidence for zoonotic transmissions of the disease to cervid hunters or consumers of meat from elk and deer.Citation6,Citation11 However, as discussed by Belay et al.Citation6 the intensity of human exposure to CWD prions may increase due to a further spread and rising prevalence of the disease in cervids. Therefore, and with the generally long latency periods of human prion diseases in mind, previous epidemiological findings cannot be readily extrapolated.
Until recently, experimental studies that pursued biochemical approaches or used transgenic mice to ascertain the susceptibility of humans to CWD infections consistently seemed to corroborate current epidemiological findings: CWD-infected cervid brain tissue did not seed the conversion of PrPC into PrPres in PMCA assays using brain homogenate from macaques or transgenic mice expressing human PrPC as test substrate,Citation12 and transgenic mice overexpressing human PrPC were resistant to infection after intracerebral challenge with CWD prions from mule deer.Citation13 However, a study published by Barria et al.Citation14 in March 2011 found that cervid PrPTSE can seed the conversion of human PrPC into PrPres by PMCA when the CWD agent has been previously passaged in vitro or in vivo. Specifically, this was demonstrated for CWD prions from naturally affected mule deer either passaged by serial PMCA using deer PrPC as conversion substrate or in transgenic mice expressing cervid PrPC. The authors of this study pointed out that CWD prions may undergo a gradual process of change and adaptation via successive passages in the cervid population. They concluded that the reported findings, if corroborated by infectivity assays, may imply “that CWD prions have the potential to infect humans and that this ability progressively increases with CWD spreading.”
Cynomolgus macaques used as a primate model for testing the susceptibility of humans to CWD as close to reality as possible have not shown clinical signs of a prion disease at nearly 6 years after intracerebral or peroral inoculation of CWD agents from white-tailed deer, Rocky Mountain elk or mule deer.Citation15 In contrast to macaques, squirrel monkeys were susceptible to CWD infection by the intracerebral route and showed even a low rate of disease transmission after oral challenge.Citation15,Citation16 Since humans are phylogenetically closer related to macaques than to squirrel monkeys, macaques are regarded as the more relevant primate model for assessing the zoonotic transmissibility of CWD.Citation15
Ongoing transmission studies in macaques.
In addition to the primate study by Race et al.Citation15 two further studies in which macaques were challenged with tissue homogenates from CWD-affected cervids by intracerebral inoculation or via the oral route have been reported to be in progress.Citation17,Citation18 The purpose, research effort, financial investment and ethical aspects of these studies demand an utmost experimental scrutiny, careful data analysis and thorough exploitation of results. This has two immediate implications: (1) Since the incubation period of CWD may be very long in case of primary (i.e., inter-species) transmission to macaques a sustained monitoring of the animals appears mandatory for many years despite negative interim findings. (2) Increasing evidence suggests the existence of different CWD agents (see below), and theoretically, CWD prions may also change over time thereby possibly altering their potential host range. Thus, CWD isolates used in individual or pooled inocula for the challenge of macaques should be typed as precisely as possible in terms of their strain characteristics and molecular identity. Other field isolates could then be checked for their similarity or dissimilarity to the macaque-tested CWD agents in order to ascertain whether or not they are covered by the ongoing primate risk assessments.
Evidence for Distinct CWD Strains
Biochemical indications for isolate-dependent structural differences of PrPTSE.
In 2002 it was reported that glycoform patterns of PrPTSE showed differences among individual CWD-affected cervids.Citation19 In a variety of studies the glycosylation of PrPTSE had been previously established as a biochemical feature that may differ between distinct TSE agents.Citation20,Citation21 Accordingly, the finding by Race et al. possibly indicated CWD infections with different or multiple strains of agent, although, alternatively, it could also be explained by random selection from a heterogeneous population of CWD-affected ruminants.Citation19
Using a conformation-dependent immunoassay (CDI), Safar et al. found evidence for different conformations of PrPTSE in elk CWD as compared with white-tailed and mule deer CWD.Citation22 However, the amino acid sequences of elk and deer PrPC differ at residues 226 (glutamic acid in elk and glutamine in deer), and it remained to be established whether the structural differences detected by CDI were related to biologically distinct CWD strains.
Isolation of CWD-associated agents causing distinct phenotypes in laboratory rodents.
Classically, prion strains are differentiated based on their incubation periods in inbred mice with distinct PrP genotypes and by lesion profiles of the vacuolation in selected brain areas of reporter animals.Citation23 When Raymond et al. serially passaged a CWD inoculum from mule deer either in Syrian hamsters or first into transgenic mice expressing hamster PrPC, and then further on in hamsters, they obtained two distinct isolates termed SghaCWDmd-f and SghaCWDmd-s, respectively.Citation24 The first isolate showed an about 5-fold shorter incubation period in Syrian hamsters than the latter, and the cerebral patterns of PrPTSE deposition and gliosis in clinically affected hamsters were also different. Based on their findings the authors concluded that the “cervid-derived inocula may have contained or diverged into at least two distinct transmissible spongiform encephalopathy strains.”
Angers et al. transmitted CWD inocula from elk and deer to transgenic mice expressing cervid PrP and found that these mice were affected by one of two strains, referred to as CWD1 and CWD2, that caused different incubation times and lesion profiles.Citation25 The results of this study “appear to reflect strain constitutions in the natural host, rather than adaptation and divergence of progenitor strains in recipient mice,” according to the authors. Interestingly, CWD1 and CWD2 did not show recognizably different biochemical properties of their PrPTSE. The electrophoretic migration and glycosylation patterns as well as the stability characteristics after treatment with guanidine hydrochloride were indistinguishable for CWD1- and CWD2-associated PrPTSE. Consistent with these findings it has been previously reported that biologically distinct prion strains cannot always be differentiated by biochemical PrPTSE-typing or characterization of the conformational stability of PrPTSE.Citation26,Citation27
Rationale for the typing of CWD agents by Fourier transform-infrared (FT-IR) spectroscopy.
The precise identification of present and newly emerging CWD strains in the field is of great importance for a reliable risk assessment. When it comes to the typing of CWD agents that have been used to challenge macaques and of other CWD field isolates for comparative purposes, a comprehensive biological characterization in rodent models would be laborious, time-consuming and, in practical respect, possibly even unfeasible. On the other hand, biochemical surrogate methods for prion typing may fail to sufficiently discriminate between different PrPTSE species associated with distinct CWD agents. The biophysical discrimination of CWD isolates by infrared spectroscopy of pathological prion protein may provide a resort from this quandary.
Infrared spectroscopy delivers information about structural characteristics of proteins.
The glycosylation and/or the folding and aggregation structure of PrPTSE mirror phenotypic characteristics of prion strains and have been also suggested as molecular codes that encipher strain-specific information of TSE agents.Citation20,Citation21,Citation26,Citation28–Citation30 Infrared spectroscopy has been established as a powerful tool for studying structural properties of proteins. Secondary structure motifs of proteins are reflected in IR spectral absorption bands, and the frequency positions of structure-sensitive amide band components can be assigned to specific types of secondary structure such as α-helix, β-sheet or turn.Citation31 The amide I band of IR spectra extends from about 1,600 to 1,700 wavenumbers per cm and primarily represents the carbonyl stretching vibration of the peptide backbone. This band is influenced by a variety of factors such as the strength of hydrogen bonds and the packing of β-strands.Citation32,Citation33
Discrimination of prion strains by infrared spectroscopy of PrPTSE.
The amide I band of IR spectra was shown in previous studies to mirror structural differences in intra- und intermolecular β-sheets, α-helices and turns of PrPTSE from different prions strains. Using Fourier transform-infrared (FT-IR) spectroscopy, Caughey et al. had been able to demonstrate that PrPTSE extracts from the brains of hamsters infected with hyper- and drowsy strains of transmissible mink encephalopathy showed different β-sheet structures.Citation30
In a later study, Thomzig et al. examined PrPTSE from three different hamster-adapted scrapie strains (263K, ME7-H, 22A-H) and a hamster-adapted BSE isolate (BSE-H) by FT-IR spectroscopy.Citation26 Two of these agents, ME7-H and 22A-H, differed only with respect to their incubation times in hamsters. Apart from that, they caused indistinguishable clinical symptoms and neuropathological lesion profiles. Differences between the electrophoretic mobilities or glycosylation patterns of their PrPTSE could not be detected. Paraffin-embedded tissue (PET) blotting of the cerebral PrPTSE distribution and protein gel blotting for testing the conformational stability of PrPTSE after exposure to Proteinase K at different pH values also failed to display discrepancies between the ME7-H and 22A-H scrapie strains. However, all four TSE agents examined in the study by Thomzig et al., including ME7-H and 22A-H, could be clearly distinguished from each other by distinct structural features of their particular PrPTSE represented in the amide I absorption patterns of FT-IR spectra.
The consistency and discriminatory significance of the spectral profiles reported by Thomzig et al. could be objectively confirmed by multivariate statistics. In a hierarchical cluster analysis the distinct amide I absorption features reliably produced four clusters of spectra, each correctly including the spectra from PrPTSE associated with one of the four different isolates.Citation34 Thus, FT-IR spectroscopy was shown to provide a “fingerprint” of β-sheet and other secondary structure components in PrPTSE that may be used for the swift typing of prion strains without restrictions to specific TSEs or host species.
Outlook
provides an outline of how CWD agents could be perspectively typed by FT-IR spectroscopy. If CWD isolates contained only single prion strains, their classification would appear theoretically feasible simply by straightforward FT-IR spectroscopical characterization of PrPTSE purified from affected cervids. However, if mixtures of different strains were present in test samples, such direct FT-IR spectroscopy of PrPTSE from affected cervids may not allow a proper typing. In principle, this potential drawback could be dispelled by the separation and cloning of agents prior to further analyses. Usually, this would have required comprehensive passages of isolates in animals.Citation23 However, due to substantial technical advancements, the PMCA technology now provides an in vitro option for the selective amplification of PrPres from distinct CWD strains.Citation5,Citation35,Citation36 For this purpose PMCA would need to be performed repeatedly with limiting dilutions of CWD seeding activity. Amplificates of PrPres derived from CWD agents by this approach could be subjected additionally or alternatively to PrPTSE from cervids to subsequent FT-IR analyses for a tentative structure-based classification of CWD agents.
We have recently found that highly-resolved FT-IR spectra for strain differentiation can be conveniently obtained from purified preparations of PMCA-derived scrapie-associated PrPres (manuscript in preparation). However, whether PrPTSE isolated from CWD-affected cervids or PrPres amplified by PMCA will eventually provide practical analytes for the typing of CWD prions by FT-IR spectroscopy requires further research. More clarity about the similarities or dissimilarities between the CWD inocula that have been administered to macaques and the variety of field isolates which cannot be tested in this way would be worth the effort.
Disclosure of Potential Conflicts of Interest
No potential conflicts of interest were disclosed.
Abbreviations
BSE | = | bovine spongiform encephalopathy |
BSE-H | = | hamster-adapted BSE agent |
CDI | = | conformation-dependent immunoassay |
CJD | = | creutzfeldt-jakob disease |
CWD | = | chronic wasting disease |
FT-IR spectroscopy | = | Fourier transform-infrared spectroscopy |
ME7-H | = | hamster-adapted scrapie agent |
PET | = | paraffin-embedded tissue |
PMCA | = | protein misfolding cyclic amplification |
PrP | = | prion protein |
PrPC | = | cellular isoform of the prion protein |
PrPres | = | protease-resistant core of misfolded prion protein |
PrPTSE | = | pathological isoform of the prion protein |
TSE | = | transmissible spongiform encephalopathy |
vCJD | = | variant creutzfeldt-jakob disease |
WTD | = | white-tailed deer |
22A-H | = | hamster-adapted scrapie agent |
263K | = | hamster-adapted scrapie agent |
Figures and Tables
Figure 1 Typing of CWD agents by FT-IR spectroscopy of strain-related misfolded prion protein. Unknown CWD field isolates may contain single or multiple strains of agent. For the typing of CWD agents by FT-IR spectroscopy purified PrPTSE from cervids affected with a single strain only could be directly examined. However, mixtures of distinct CWD agents would need to be separated into single strains prior to FT-IR spectroscopic analysis. Perspectively, this may be achieved by PMCA repeatedly performed with limiting dilutions of CWD seeding activity (limiting dilutions would be identified as the highest dilutions of CWD test samples that still show a detectable seeding activity, i.e., formation of PrPres). If a test sample contained two CWD strains, A and B, and the seeding activity of strain A exceeded that of strain B under specific PMCA conditions, PMCA performed with limiting dilutions should selectively amplify PrPres derived from strain A. Vice versa, PrPres derived from strain B should be selectively amplified if the seeding activity of this strain exceeded that of strain A. Thus, amplification of PrPres from the CWD strain with the relatively highest seeding activity should be achieved by the depicted analytical approach. It has to be noted that the relative seeding activities of different strains in a given CWD isolate (1) do not need to quantitatively correlate with the relative amounts of their respective PrPTSE and (2) may depend on the specific PMCA conditions used for PrPres amplification. Therefore, if aliquots of a CWD isolate were processed under differing PMCA conditions favoring the amplification of PrPres from different strains, this could potentially allow the isolation of PrPres amplificates from two or more CWD strains in the sample. Once highly purified PrPTSE extracts or PrPres amplificates have been prepared, these can be subjected to FT-IR spectroscopy. The obtained spectral information comprised in the amide I absorption band between about 1,600–1,700 wavenumbers/cm should provide a structural fingerprint of β-sheets (low frequency, a), unassigned structure (b), α-helices (c), turns (d) and turns/β-sheets (high frequency, e) of the examined PrPTSE/PrPres as shown in the FT-IR second derivative spectrum of the figure. This spectral information can be used as input data for a hierarchical cluster analysis in order to obtain an objective sample classification in terms of tentative CWD strain assignments.Citation26,Citation34 Abbreviations: A, CWD strain A; B, CWD strain B. Symbols: circles, misfolded prion protein associated with or PMCA-derived from CWD strain A; triangles, misfolded prion protein associated with or PMCA-derived from CWD strain B.
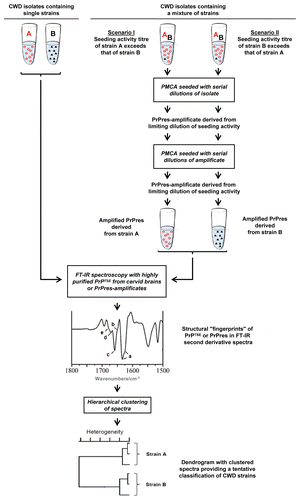
Acknowledgments
We would like to thank the Alberta Prion Research Institute (Canada) for financial support of studies in our laboratory aiming at the detection, typing and risk assessment of prions causing chronic wasting disease (Project “Comprehensive risk assessment of CWD transmission to humans using non-human primates”; Coordinator: Dr. Stefanie Czub, Canadian Food Inspection Agency, Lethbridge/Alberta, Canada). We are also grateful to Dieter Naumann and Peter Lasch (P25-Biomedical Spectroscopy, Robert Koch-Institut, Berlin, Germany) for long-standing cooperation in the field of FT-IR spectroscopy, and to Achim Thomzig (P24-Transmissible Spongiform Encephalopathies, Robert Koch-Institut, Berlin, Germany) for critical discussion of the manuscript.
Funding
The Alberta Prion Research Institute (Canada) financially supports work of the authors aiming at the detection, typing and risk assessment of prions causing chronic wasting disease (Project “Comprehensive risk assessment of CWD transmission to humans using non-human primates”). The funders had no role in the decision to publish or in the preparation of the manuscript.
References
- Prusiner SB. Prions. Proc Natl Acad Sci USA 1998; 95:13363 - 13383; PMID: 9811807; http://dx.doi.org/10.1073/pnas.95.23.13363
- Collee JG, Bradley R, Liberski PP. Variant CJD (vCJD) and Bovine Spongiform Encephalopathy (BSE): 10 and 20 years on: part 2. Folia Neuropathol 2006; 44:102 - 110; PMID: 16823692
- Brown P, Cervenakova L. A prion lexicon (out of control). Lancet 2005; 365:122; PMID: 15639292; http://dx.doi.org/10.1016/S0140-6736(05)17700-9
- Soto C. Prion hypothesis: the end of the controversy?. Trends Biochem Sci 2011; 36:151 - 158; PMID: 21130657; http://dx.doi.org/10.1016/j.tibs.2010.11.001
- Castilla J, Saa P, Morales R, Abid K, Maundrell K, Soto C. Protein misfolding cyclic amplification for diagnosis and prion propagation studies. Methods Enzymol 2006; 412:3 - 21; PMID: 17046648; http://dx.doi.org/10.1016/S0076-6879(06)12001-7
- Belay ED, Maddox RA, Williams ES, Miller MW, Gambetti P, Schonberger LB. Chronic wasting disease and potential transmission to humans. Emerg Infect Dis 2004; 10:977 - 984; PMID: 15207045
- Gilch S, Chitoor N, Taguchi Y, Stuart M, Jewell JE, Schätzl HM. Chronic wasting disease. Top Curr Chem 2011; Epub ahead of print http://dx.doi.org/10.1007/128_2011_159
- Angers RC, Browning SR, Seward TS, Sigurdson CJ, Miller MW, Hoover EA, et al. Prions in skeletal muscles of deer with chronic wasting disease. Science 2006; 311:1117; PMID: 16439622; http://dx.doi.org/10.1126/science.1122864
- Angers RC, Seward TS, Napier D, Green M, Hoover E, Spraker T, et al. Chronic wasting disease prions in elk and antler velvet. Emerg Infect Dis 2009; 15:696 - 703; PMID: 19402954; http://dx.doi.org/10.3201/eid1505.081458
- Daus ML, Breyer J, Wagenfuehr K, Wemheuer WM, Thomzig A, Schulz-Schaeffer WJ, et al. Presence and seeding activity of pathological prion protein (PrPTSE) in skeletal muscles of white-tailed deer infected with chronic wasting disease. PLoS ONE 2011; 6:18345; PMID: 21483771; http://dx.doi.org/10.1371/journal.pone.0018345
- Belay ED, Abrams J, Kenfield J, Weidenbach K, Maddox RA, Lawaczeck E, et al. Monitoring the potential transmission of chronic wasting disease to humans (Abstract Oral.40, Prion 2011 Oral Presentations). Prion 2011; 5:17 Supplemental Issue April/May/June 2011
- Kurt TD, Telling GC, Zabel MD, Hoover EA. Trans-species amplification of PrP(CWD) and correlation with rigid loop 170N. Virology 2009; 387:235 - 243; PMID: 19269662; http://dx.doi.org/10.1016/j.virol.2009.02.025
- Sandberg MK, Al-Doujaily H, Sigurdson CJ, Glatzel M, O'Malley C, Powell C, et al. Chronic wasting disease prions are not transmissible to transgenic mice overexpressing human prion protein. J Gen Virol 2010; 91:2651 - 2657; PMID: 20610667; http://dx.doi.org/10.1099/vir.0.024380-0
- Barria MA, Telling GC, Gambetti P, Mastrianni JA, Soto C. Generation of a new form of human PrPSc in vitro by interspecies transmission from cervid prions. J Biol Chem 2011; 286:7490 - 7495; PMID: 21209079; http://dx.doi.org/10.1074/jbc.M110.198465
- Race B, Meade-White KD, Miller MW, Barbian KD, Rubenstein R, LaFauci G, et al. Susceptibilities of nonhuman primates to chronic wasting disease. Emerg Infect Dis 2009; 15:1366 - 1376; PMID: 19788803; http://dx.doi.org/10.3201/eid1509.090253
- Marsh RF, Kincaid AE, Bessen RA, Bartz JC. Interspecies transmission of chronic wasting disease prions to squirrel monkeys (Saimiri sciureus). J Virol 2005; 79:13794 - 13796; PMID: 16227298; http://dx.doi.org/10.1128/JVI.79.21.13794-6.2005
- Comoy E, Durand V, Correia E, Balachandran A, Richt JA, Beringue V, et al. Zoonotic potential of CWD: Experimental transmissions to non-human primates (Abstract Envt.06, Prion 2011 Poster Presentations). Prion 2011; 5:101
- Motzkus D, Schulz-Schaeffer WJ, Beekes M, Schätzl HM, Jirik FR, Schmädicke AC, et al. Transmission of CWD to non-human primates: Interim results of a comprehensive study on the transmissibility to humans (Abstract Envt. 22, Prion 2011 Poster Presentations). Prion 2011; 5:107 Supplemental Issue April/May/June 2011
- Race RE, Raines A, Baron TG, Miller MW, Jenny A, Williams ES. Comparison of abnormal prion protein glycoform patterns from transmissible spongiform encephalopathy agent-infected deer, elk, sheep and cattle. J Virol 2002; 76:12365 - 12368; PMID: 12414979; http://dx.doi.org/10.1128/JVI.76.23.12365-8.2002
- Parchi P, Capellari S, Chen SG, Petersen RB, Gambetti P, Kopp N, et al. Typing prion isoforms. Nature 1997; 386:232 - 234; PMID: 9069279; http://dx.doi.org/10.1038/386232a0
- Aguzzi A, Heikenwalder M, Polymenidou M. Insights into prion strains and neurotoxicity. Nat Rev Mol Cell Biol 2007; 8:552 - 561; PMID: 17585315; http://dx.doi.org/10.1038/nrm2204
- Safar JG, Scott M, Monaghan J, Deering C, Didorenko S, Vergara J, et al. Measuring prions causing bovine spongiform encephalopathy or chronic wasting disease by immunoassays and transgenic mice. Nat Biotechnol 2002; 20:1147 - 1150; PMID: 12389035; http://dx.doi.org/10.1038/nbt748
- Bruce ME, Fraser H. Scrapie strain variation and its implications. Curr Top Microbiol Immunol 1991; 172:125 - 138; PMID: 1810707
- Raymond GJ, Raymond LD, Meade-White KD, Hughson AG, Favara C, Gardner D, et al. Transmission and adaptation of chronic wasting disease to hamsters and transgenic mice: evidence for strains. J Virol 2007; 81:4305 - 4314; PMID: 17287284; http://dx.doi.org/10.1128/JVI.02474-06
- Angers RC, Kang HE, Napier D, Browning S, Seward T, Mathiason C, et al. Prion strain mutation determined by prion protein conformational compatibility and primary structure. Science 2010; 328:1154 - 1158; PMID: 20466881; http://dx.doi.org/10.1126/science.1187107
- Thomzig A, Spassov S, Friedrich M, Naumann D, Beekes M. Discriminating scrapie and bovine spongiform encephalopathy isolates by infrared spectroscopy of pathological prion protein. J Biol Chem 2004; 279:33847 - 33854; PMID: 15155741; http://dx.doi.org/10.1074/jbc.M403730200
- Peretz D, Scott MR, Groth D, Williamson RA, Burton DR, Cohen FE, et al. Strain-specified relative conformational stability of the scrapie prion protein. Protein Sci 2001; 10:854 - 863; PMID: 11274476; http://dx.doi.org/10.1110/ps.39201
- Bessen RA, Kocisko DA, Raymond GJ, Nandan S, Lansbury PT, Caughey B. Non-genetic propagation of strain-specific properties of scrapie prion protein. Nature 1995; 375:698 - 700; PMID: 7791905; http://dx.doi.org/10.1038/375698a0
- Telling GC, Parchi P, DeArmond SJ, Cortelli P, Montagna P, Gabizon R, et al. Evidence for the conformation of the pathologic isoform of the prion protein enciphering and propagating prion diversity. Science 1996; 274:2079 - 2082; PMID: 8953038; http://dx.doi.org/10.1126/science.274.5295.2079
- Caughey B, Raymond GJ, Bessen RA. Strain-dependent differences in beta-sheet conformations of abnormal prion protein. J Biol Chem 1998; 273:32230 - 32235; PMID: 9822701; http://dx.doi.org/10.1074/jbc.273.48.32230
- Fabian H, Mäntele W. Chalmers JM, Griffiths PR. Infrared spectroscopy of proteins. Handbook of Vibrational Spectroscopy 2002; Chichester, UK John Wiley & Sons 3399 - 3425
- Surewicz WK, Mantsch HH. New insight into protein secondary structure from resolution-enhanced infrared spectra. Biochim Biophys Acta 1988; 952:115 - 130; PMID: 3276352; http://dx.doi.org/10.1016/0167-4838(88)90107-0
- Zandomeneghi G, Krebs MRH, McCammon MG, Fandrich M. FTIR reveals structural differences between native-sheet proteins and amyloid fibrils. Protein Sci 2004; 13:3314 - 3321; http://dx.doi.org/10.1110/ps.041024904
- Spassov S, Beekes M, Naumann D. Structural differences between TSEs strains investigated by FT-IR spectroscopy. Biochim Biophys Acta 2006; 1760:1138 - 1149; PMID: 16730908; http://dx.doi.org/10.1016/j.bbagen.2006.02.018
- Gonzalez-Montalban N, Makarava N, Ostapchenko VG, Savtchenk R, Alexeeva I, Rohwer RG, et al. Highly efficient protein misfolding cyclic amplification. PLoS Pathog 2011; 7:1001277; PMID: 21347353; http://dx.doi.org/10.1371/journal.ppat.1001277
- Pritzkow S, Wagenfuhr K, Daus ML, Boerner S, Lemmer K, Thomzig A, et al. Quantitative detection and biological propagation of scrapie seeding activity in vitro facilitate use of prions as model pathogens for disinfection. PLoS ONE 2011; 6:20384; PMID: 21647368; http://dx.doi.org/10.1371/journal.pone.0020384