Abstract
As compared with plant system, triose phosphate isomerase (TPI), a crucial enzyme of glycolysis, has been well studied in animals. In order to characterize TPI in plants, a full-length cDNA encoding OscTPI was cloned from rice and expressed in E. coli. The recombinant OscTPI was purified to homogeneity and it showed Km value of 0.1281 ± 0.025 µM, and the Vmax value of 138.7 ± 16 µmol min−1mg−1 which is comparable to the kinetic values studied in other plants. The OscTPI was found to be exclusively present in the cytoplasm when checked with the various methods. Functional assay showed that OscTPI could complement a TPI mutation in yeast. Real time PCR analysis revealed that OscTPI transcript level was regulated in response to various abiotic stresses. Interestingly, it was highly induced under different concentration of methylglyoxal (MG) stress in a concentration dependent manner. There was also a corresponding increase in the protein and the enzyme activity of OscTPI both in shoot and root tissues under MG stress. Our result shows that increases in MG leads to the increase in TPI which results in decrease of DHAP and consequently decrease in the level of toxic MG.
Introduction
Methylglyoxal (MG), a cytotoxin, is a typical 2-oxoaldehyde byproduct of several metabolic pathways such as threonine catabolism and lipid peroxidation. However, the major source of production of MG is via glycolysis where it is produced from non-enzymatic elimination of phosphate from glyceraldehyde-3-phosphate (GAP) and dihydroxyacetone phosphate DHAP. The interconversion of DHAP and GAP is catalyzed by a crucial glycolytic enzyme, triose phosphate isomerase (TPI) which plays an important role in other metabolic pathways involving triosephosphate, such as gluconeogenesis, fatty acid biosynthesis, pentosephosphate pathway, and photosynthetic carbon dioxide fixation.Citation1 High resolution crystal structures are available for TPI from chicken,Citation2 yeast,Citation3 and Trypanosoma brucei.Citation4 The extremely high turnover rate of TPI has led to its description as a nearly perfect catalyst.Citation5 Genes encoding TPI have been cloned and sequenced from 11 species, including chicken,Citation6 humanCitation7,Citation8 maize,Citation9 mouse,Citation10 Aspergillus nidulans,Citation11 Coptis japonica,Citation12 Escherichia coli,Citation13 Macaca mulatta,Citation14 T. brucei,Citation15 Saccharomyces cerevisiae,Citation16 and Schizosaccharomyces pombe.Citation17 The high degree of sequence conservation of TPI suggests a low rate of evolutionary divergence, a common feature of all the glycolytic enzymes.Citation18 Isolation and characterization of a cDNA clone encoding the cytosolic form of TPI from rice has also been reported.Citation19
It has been reported that loss of TPI activity results in elevated levels of DHAPCitation20,Citation21 which in turn, is spontaneously converted to MG that modifies proteins and DNA through the formation of Advanced Glycation End products (AGEs) are deleterious to cells.Citation22 Enhanced AGE production also leads to many disease symptoms in humans.Citation23 However, under conditions of oxidative stress, glutathione levels are reduced, resulting in elevated levels of MG which, in turn, lead to progressive neurological dysfunction, neurodegeneration, and early death. This effect can be further magnified in a positive feedback loop because AGEs themselves contribute to the production of reactive oxygen species.Citation24
Although MG is synthesized during normal metabolism of glucose, it inhibits the growth of cells of microorganisms as well as higher eukaryotes, eventually killing them. Therefore, studies on the metabolic effect of MG are of considerable interest. It has been earlier reported that MG is metabolized to lactic acid by two different routes.Citation25 One is the glyoxalase system in which glyoxalase I and glyoxalase II are involved. In this route, MG is condensed with glutathione to give S-D-lactoylglutathione by the action of glyoxalase I, and the glutathione thiolester is then hydrolyzed to lactic acid and glutathione by glyoxalase II.Citation23 Glyoxalase pathway in plants have also been reported as a major detoxification pathway in plants.Citation26 The other route is a reduction/oxidation system consisting of methylglyoxal reductase and lactaldehyde dehydrogenase. MG is reduced to lactaldehyde by NADPH dependent methylglyoxal reductase, and lactaldehyde is further oxidized to lactic acid by NAD+-dependent lactaldehyde dehydrogenase.Citation27
There is an abundance of literature on the role, structure and regulation of animal TPIs. However, the current research on plant TPI is limited. Molecular weight of TPI has been depicted in rabbit,Citation28 yeast,Citation16 and bacteriaCitation29 which is found to be 247 to 255 amino acids long polypeptide chains of approximate molecular weight of 27,000 Daltons. In vascular plants, only a limited number of studies have been undertaken to show the function of TPI where it was first purified from pea seeds.Citation30 The existence of plastid and cytosolic TPI isozymes was first reported in an extensive survey of isoelectric points of the enzyme in numerous species of angiosperms, gymnosperms, ferns, and fern allies.Citation31 TPI isozyme has also been purified from spinach, lettuce, and celery.Citation13
In the present study, we report that rice cytoplasmic TPI is induced in response to MG stress.MG affects the transcript, protein and enzyme activity. This could be a mechanism to bring down the toxic levels of MG under stress conditions defending the plants against abiotic stress.
Results
Identification and chromosomal distribution of TPI genes in rice
We have identified 3 TPI genes within the rice genome (TIGR, version 6.1), encoding three isoforms of TPI proteins. They have been demarcated on the basis of cellular localization of the encoded proteins. The physical distribution of TPI genes on different chromosomes of rice is shown in . Out of three isoforms, two are located on chromosome no I, while the third is situated on chromosome IX. The genes on chromosome I encode cytosolic proteins and show about 70% homology at amino acid level with each other (). The gene located on chromosome IX encodes proteins localized in chloroplast. A chloroplastic isoform of triose phosphate isomerase (pdTPI) has been recently found important in the postgerminative seedling establishment and it also plays a crucial role in the transition from heterotrophic to autotrophic growth to provide energy and carbon in Arabidopsis.Citation32
Figure 1. Chromosomal distribution events for TPI genes. (A) Three genes for TPI located on chromosome I and IX in rice. The scale is in megabase (Mb), and the centromeric regions are indicated by ellipses. (B) Multiple alignment of TPI genes in rice where first two sequences show the cytosolic TPI and the last one represents the chloroplastic TPI. ClustalW2 program was used to generate the alignment. (C) Unrooted tree generated for cTPI from different organisms using neighbor joining method employing Mega4 software. (D) Expression profile of TPI genes from Affymetrix GeneChip Rice Genome Arrays (http://www.ncbi.nlm.nih.gov/geo). The heatmaps have been generated using TIGR MeV software package. The color bar represents relative expression values; thereby, green color represents lowest expression levels, black represents medium expression levels, and red signifies highest expression level
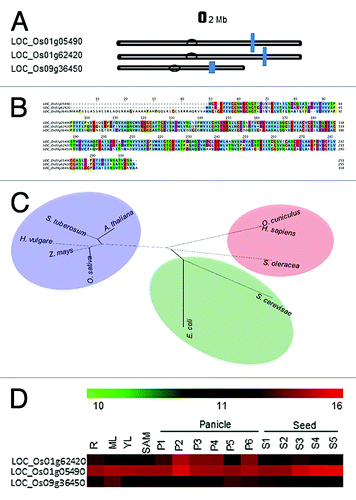
Phylogenetic tree reveals that there is about 60–80% similarity between the TPI sequences of Arabidopsis thaliana, Solanum tuberosum, Hordeum vulgare, Zea mays and Oryza sativa ().
The cytoplasmic TPI, present on the chromosome no I (LOC_Os01g62420) was selected for further studies, which showed four splice forms (http://rice.plantbiology.msu.edu/). The CDS coordinates (5′-3′) is from 36129310 – 36132720. The predicted nucleotide and amino acid length is 768 bp and 255 aa respectively, whereas the molecular weight is 27273.4 D.
For microarray analysis of rice TPI genes, Affymetrix GeneChip Rice Genome Arrays (http://www.ncbi.nlm.nih.gov/geo/) was used. Spatial and temporal gene expression in various tissues/organs and developmental stages of rice was analyzed to identify the genes differentially expressed during various stages of reproductive development. OscTPI (LOC_Os01 g62420) was interestingly expressed at all the stages of development as compared with the other isoforms ()
Expression, purification and specific activity of recombinant OscTPI
Clone of rice TPI located on chromosome no I was amplified and further cloned into pET28a bacterial expression vector. The pET28aOscTPI recombinant plasmid was transformed into BL21 (DE3) a specific host strain of E. coli, for expression of the recombinant protein. A highly induced band of 27 kDa was visible on SDS-PAGE, which was not present in the uninduced cells as well as induced negative control (BL21 cells containing empty vector (). The recombinant protein was purified by anion exchange chromatography and monitored on SDS-PAGE (). The eluted purified protein fractions were pooled and dialyzed against 20 mM Hepes buffer pH 8.0. Yield of the eluted soluble protein was about 7.0 mg/ml of culture.
Figure 2. Expression, purification and Kinetic parameters for OscTPI. (A) Heterologous expression of OscTPI recombinant protein in bacterial expression host E. coli BL21 (DE3) cells: Coomassie stained PAGE showing crude protein extract from untransformed (empty vector), uninduced and OscTPI transformed BL21 (DE3) cells induced at 37°C with 0.1 mM of IPTG. (B) Purification of recombinant OscTPI. Coomassie stained PAGE showing Ni-NTA affinity purified recombinant OsTPI uninduced and purified protein (1–3 Elution fractions in 250 mM imidazole). (C) Determination of kinetic parameters of OscTPI: Extrapolation of Km and Vmax values were done using Lineweaver-burk plot
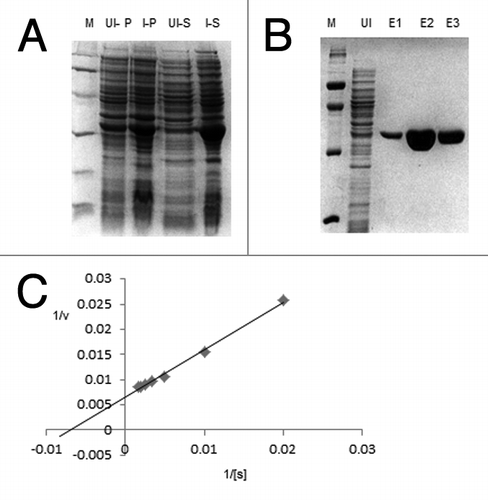
Enzyme kinetics of OscTPI
Different kinetic parameters such as Km and Vmax were determined with purified recombinant OscTPI by systematically varying the concentration of glyceraldehyde 3 phosphate (50 µM to 1 mM) in the presence of NADH. The Km was found to be 0.1281 µM, and the Vmax 138.7 µmol min−1mg−1 (). The kinetic values were very similar to other TPIs of many organisms such as Potato, Yeast, Rabbit and Chicken muscle.Citation33 ()
Table 1. Primer sequences used for cloning and Real Time PCR
Functional complementation of yeast TPI (TIM1) mutant with OscTPI
To confirm whether the OscTPI can functionally complement the yeast TIM1 (YDR050C) mutant by reverting the sensitive phenotype, OscTPI was cloned in yeast expression vector pYES2 under pGAL1 promoter which is galactose inducible and glucose repressible. S. cerevisiae strain BY4741 and TPI deletion strain in the BY4741 background were transformed with pYES2OscTPI construct or empty vector (pYES2 plasmid). Since pYES2 vector has Ura+ as the selection marker, the transformed yeast cells were grown on Ura- SD media containing either glucose or galactose at 37°C while TIM mutant with empty vector was unable to grow at 37°C, the BY4741 WT yeast strain could grow. However, when the TIM mutant was transformed with pYES2OscTPIconstruct, it was able to complement the growth defect of TIM1 mutant at 37°C (in galactose containing media). This result clearly indicates that the rice OscTPI can functionally complement TIM mutation in yeast ().
Localization of TPI
To verify the cellular location, OscTPI cDNA was cloned into a GFP based vector, pMBPII-GFP. In the resulting plasmid pMBGFP-TPI, the expression of gene is governed by the strong constitutive cauliflower mosaic virus 35S promoter (CaMV 35S) and the nopaline synthase terminator (NOS-T). Upon inspection of Nicotiana tabacum protoplast transiently transfected with this plasmid, the protein was localized in the cytosol, and evenly distributed without forming any visible aggregates (). The fluorescence was not detected in the untransfected control cells, confirming that the green fluorescence indeed originated from the expression of the introduced pMBGFP-OscTPI.
Figure 4. Localization of OscTPI. (A) The expression of OscTPI –GFP fused protein in tobacco mesophyll protoplast. Tobacco protoplsts were transiently transformed by PEG transfection using TPI-pMB construct (B) Onion peel epidermal cells showing expression of the OscTPI - green fluorescent (GFP) fusion protein driven by the 35S promoter, showing its distribution throughout the cytoplasm. (C) Cellular fractionation and Western Blot Hybridization
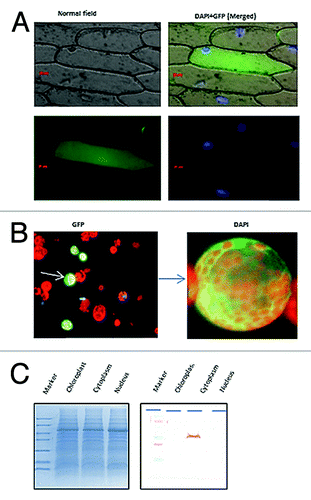
Localization assay for OscTPI was also performed using onion peel bombardment assay (). The ORF of OscTPI was cloned into pMB-GFP vector and pMBGFP-OscTPI construct was used for onion peel transformation by particle bombardment using the PDS-1000 particle delivery system (Bio-Rad, USA). When observed under fluorescence microscope at 488 nm-exciting wavelengths, it was found to be equally distributed in the cytoplasm.
To further confirm the localization of TPI, cellular fractionation from 12 d old rice seedlings was performed by ultracentrifugation and various fractions such as cytosol, chloroplast and nuclei was collected. All the three fractions were loaded on SDS-PAGE and western blot hybridization was performed using the antibodies raised against TPI which reconfirmed the cytoplasmic nature of TPI.
Transcript analysis of OscTPI in response to various stresses
In order to understand the response of the cytoplasmic TPI under various stresses at different time points, the relative transcript level of OscTPI was determined by quantitative RT-PCR after exposure of 6 h and 24 h in response to high temperature (42°C), drought (RT), wounding, low temperature (4°C), salt stress (NaCl, 200 mM), oxidative stress (H2O2, 5 mM), and methylglyoxal (5 mM). The OscTPI transcript was about 2 folds upregulated at 6 h and 24 h following wounding and MG stress. About 1.5 folds upregulation of OscTPI was observed under low temperature and H2O2 stress at 6 h, the transcript level dramatically decreased to 0.3 folds when stress persisted for 24 h. Since relative expression ratio of OscTPI was less than 1, it showed a consistent 0.6–0.8 fold downregulation in case of heat, drought and NaCl after 6 h and 24 h after treatment ().
Figure 5. Real-Time PCR analysis to confirm transcript response. (A) Real-time PCR analysis for transcript of cytosolic TPI gene in response to different abiotic stresses in rice (Oryza sativa L. cv IR64). The analysis was done with cDNA template generated from (A) shoot tissue of 12 d old stressed or control seedlings for 6 h and 24 h and (B) 12 d old seedlings subjected to various concentration of MG for 24 h. The bars above or below 1 shows the transcript upregulation and downregulation, respectively
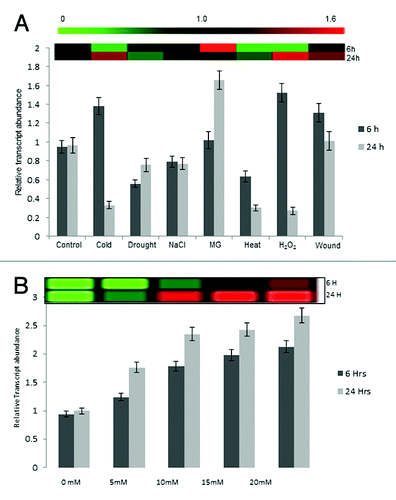
Transcript level for OscTPI were also measured in the12 d old rice seedlings given various MG stress (0, 5, 10, 15 and 20 mM) for 24 h () and transcript level was found upregulated increasing concentration of MG.
In planta enzyme activity of OscTPI under various stresses
As TPI shows higher transcript level under stress, we checked if this results in the higher enzyme activity. The specific activity was measured under similar conditions of stress treatment as given for measuring the transcript level in rice seedlings. Both root and shoot tissues were harvested after 6 and 24 h of treatment and native TPI activity was monitored. It was found that TPI activity was higher in shoots under MG, wounding and salt stress at both the time points. However, under oxidative stress, higher TPI activity was observed after 6 h which decreased by 24 h. It was also noticed that enzyme activity declined marginally at both the time points when seedlings were subjected to other stresses such as low temperature, drought and heat. In case of MG stress, it showed a good correlation with the transcript level (). In case of roots also, similar pattern of TPI activity was observed with higher activity under MG, wounding and NaCl stress while the enzyme activity declined in case of cold, drought and heat stress ().
Figure 6. Determination of specific activity of the native OscTPI under MG and other stresses. Rice seedlings were subjected to various stresses, for 6 and 24h. Specific activities were measured using crude proteins (A) 12 d old shoot. (B) 12 d old root (C) Specific activity under various concentration of MG (D) western blot hybridization assay using crude protein extracted from shoot under different MG concentration (E) Activity staining assay for OscTPI where TPI activity bands appear dark over a fluorescent background.
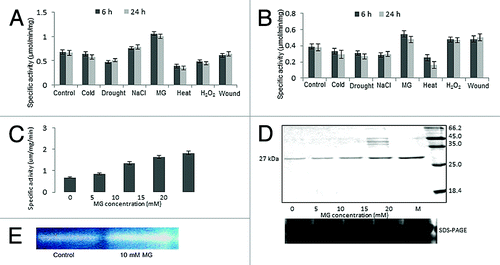
To confirm the effect of MG, TPI activity was measured using 12 d old rice seedlings exposed to different concentrations of MG for 24 h. We found that activity linearly increased with the increase of MG concentration (). The TPI activity assay was also performed on native PAGECitation34 using total protein isolated from non-stressed and MG stressed shoot tissues () which reconfirmed the higher activity of TPI under MG stress as compared with control.
To check if the increase in the enzyme activity is due to the increase in protein level, western blot was performed using the same tissues from which activity was measured. Data given in shows an increase in overall protein level with an increase in MG concentration.
Discussion
TPI, a glycolytic enzyme, is well studied in animal systems. However, not much is known about its role in plants. Earlier cytoplasmic TPI (cTPI) has been particularly characterized and purified from Solanum chacoenseCitation34 and Spinach oleracea.Citation35 In this work, we report biochemical characterization of cytoplasmic TPI from rice and its regulation under abiotic stress.
The rice TPI located on chromosome I was cloned and characterized. Amino acid sequences of TPIs from various organisms were found highly conserved and showed about 60–80% homology to plants such Zea mays, Arabidopsis thaliana, Hordeum vulgare and Solanum tuberosum. We also found by Complementation assay that rice TPI can complement the yeast TPI partially. This partial complementation could be because the protein sequence for both the organisms shares only 55–60% homology. However, the kinetic properties of OscTPI values nearly similar to other cTPIs from plants such as Solanum chacoenseCitation33 and Spinach oleracea.Citation35 The values nevertheless are lower than the Km of TPI from Homosapiens sapiens,Citation36,Citation37 Oryctolagus cuniculusCitation38 and Saccharomyces cerevisae.Citation39 A low Km value means that the enzyme has a high affinity for the substrate i.e., less concentration of substrate is enough to run the reaction at half its max speed. TPI is a non-allosteric glycolytic enzyme which performs isomerization of DHAP and GAP at pH 8.Citation40 It has been reported that the key component of the active site of TPI is the catalytic base (Glu 167) as well as electrostatic stabilization.Citation41 It has also been reported that TPI is a very efficient enzyme.Citation42 For example, the rate of conversion of D-GAP-to-DHAP is diffusion controlled; meaning that kcat/Km in this direction is close to its maximum possible value of 109 M-Citation1 s-.Citation1
Previous reports have shown that TPI in plants occurs in the cytosol and in the plastid fractionCitation43,Citation44 In fact both the isoforms have been separated and studied in pea,Citation44 spinach, lettuce, celery, and broccoli.Citation13 To confirm that the OscTPI is cytoplasmic in nature, localization assay was performed using the PEG mediated protoplast transfection which clearly indicated that it is evenly distributed in the cytosol. Localization of OscTPI was also confirmed using onion peel bombardment assay and fluorescence microscopy. We also performed cellular fractionation from rice seedlings and reconfirmed the cytoplasmic nature of OscTPI by western blot hybridization.
Rice cytosolic TPI has been reported to show higher transcript level under submergenceCitation45 and salt stress.Citation46 Most of the other glycolytic enzymes also show higher activity under hypoxia and anoxia stress in rice.Citation47 Even water stress induces TPI in maizeCitation48while no significant change has been observed in activity of TPI under salt stress in pea.Citation49 In the present study, we did not see significant upregulation in TPI transcript under NaCl (200 mM) stress. This difference could be due to the use of rice seedlings at different age, stress treatment schedule and the genotype etc. (As we have used IR64, a salt sensitive variety while Kawasaki et al. used Pokkali, which is a salt tolerant variety) under different conditions.
Our data confirms the upregulation of OscTPI under wound and MG stress, however, it was most prominent in case of MG where about 2-folds increase in the transcript level at 6 and 24 h of stress was recorded (). Interestingly, when transcript was checked from the rice seedling given various MG stress, TPI showed increase in transcript level from 0 mM to 20 mM MG (). Earlier, cytoplasmic TPI in yeast is reported to increase 7-folds under MG stress.Citation50 In humans also, it has been shown that MG causes a significant increase in transcript and protein expression of the nervous growth factor (NGF) as well as of the pro-inflammatory cytokine IL-1β, a major inducer of the acute phase response.Citation51 Though we could not find any reports where MG induces TPI particularly, it has been shown that MG induced a dose- and time-dependent increase in aldose reductase (AR) mRNA level in rat aortic smooth muscle cells.Citation52
Western blot hybridization and enzyme activity of TPI also showed a linear correlation between the TPI transcripts, protein level and enzyme activity under MG stress. This correlation was also observed under drought, heat and wound stress. However, in case of salinity stress, a downregulation was observed at the transcript level while the TPI enzyme activity was found to be slightly higher. The difference between transcript level and the enzyme activity is possibly due to the post-translational modification. Studies have shown that the correlation between protein and transcript expression levels can vary significantly based upon the profiling approach used and the system being analyzed.Citation53 Many specific examples of discordant protein/ transcript expression patterns have also been detected recently revealing examples of posttranslational regulation.Citation54
We have previously shown that in response to stress, there is an increase in MG levelCitation25 which is quite deleterious to plant growth. Our earlier studies also shows that seed germination, shoot and root growth is severely affected by exogenous application of MG. Inhibitory effect of exogenous MG on seed germination in barley has also been demonstrated in 1970s by Mankikar and Rangekar.Citation55 Our present data now indicates that there is an upregulation of TPI transcript, protein and enzyme activity under various concentration of MG. We perceive that in order to rescue the stress, the increase in MG activates the TPI which shifts the reaction toward the formation of GAP, thus decreasing the concentration of DHAP. Once DHAP level goes down, the amount of MG present in the system will decrease and hence the plant could survive under stress. Higher level of MG has been previously shown to induce several glycolytic enzymes such as, phosphoglucose isomerase (PGI), phosphofructokinase (PFK) and triosephosphate isomerase (TPI) in yeast.Citation50 It is also possible that due to increase in TPI, the amount of GAP will increase in the system which can positively affect the energy level in plant and thus support the plant survival.
Our present results elucidate that MG could act as a signal molecule to induce the expression of TPI in rice. The increase of TPI under MG stress, on one hand, decreases the level of MG by feedback mechanism, and on the other hand increases the concentration of GAP, and thus increasing the energy status of the plant against the stress consequences. This could be one of the mechanisms to defend the plant system from abiotic streses.
Materials and Methods
Genomic distribution of TPI genes on rice chromosomes
Position of each of the TPI genes on rice chromosome pseudomolecules available at TIGR version 6.1 (http://rice.plantbiology.msu.edu/cgi-bin/ORF_infopage.cgi) was determined by BLASTn search.
Multiple alignment and phylogenetic analyses of TPI
Multiple alignments of all the isoforms of rice TPI proteins were performed using ClustalW2 program.Citation56 For phylogenetic analysis, sequence from different organisms such as Arabidopsis thaliana, Solanum tubereosum, Hordeum vulgare, Zea mays Oryza sativa, Oryctolagus cuniculus, Homosapiens sapiens, Saccharomyces cerevisiae and Escherichia coli were taken and unrooted tree was generated for cytoplasmic TPI using neighbor joining method employing Mega4 software.
Expression analysis using Rice Genome database
For microarray analysis of rice TPI genes, Gene Expression Omnibus platform (http://www.ncbi.nlm.nih.gov/geo/) accession number GSE6893 was used and heatmap was generated using the Institute for Genomic Research MeV software package. Here, different stages of panicle and seed development have been categorized according to panicle length and days after pollination, respectively, based on landmark developmental events as follows: up to 0.5 mM, shoot apical meristem and rachis meristem (SAM); 0–3 cm, floral transition and floral organ development (P1); 3–10 cm, meiotic stage (P2 and P3); 10–15 cm, young microspore stage (P4); 15–22 cm, vacuolated pollen stage (P5); 22–30 cm, mature pollen stage (P6); 0–2 DAP, early globular embryo (S1); 3–4 DAP, middle and late globular embryo (S2); 5–10 DAP, embryo morphogenesis (S3); 11–20 DAP, embryo maturation (S4); 21–29, dormancy and desiccation tolerance (S5).Citation57
Plant material, growth conditions and sample collection
Plants of rice cultivar IR64 were grown in controlled conditions in growth chamber at 28 ± 2°C and 16 h photoperiod. The seeds were surface sterilized with 1% Bavistin for 20 min and allowed to germinate hydroponically with modified Yoshida medium.Citation58 Various stress treatments including low temperature (4°C), Drought, Sodium chloride (200 mm), MG (5 mM), High temperature (42°C), H2O2 and wound were given to the 12 d old plant seedlings and tissues were harvested after 6 and 24 h of stress treatment.After 7 d, various chemical treatments (prepared in Yoshida medium) were given. At specific time point, plant tissues were excised, weighed and packed in aluminum foil, labeled properly and then stored in liquid nitrogen till further use. Untreated seedlings served as control.
Cloning of OscTPI from rice
TPI gene was amplified using UTR specific primers (TIGR 6.1) () from rice. It was further cloned into TOPO-TA cloning vector (Invitrogen) and sequenced. The full length OscTPI cDNA was amplified with gene specific primers and cloned at BamH1/ Xho1 sites in pET28(a) (Novagen) bacterial expression vector.
Table 2. Km values for TPI in various organisms
For localization assay, the OscTPI cDNA was cloned in pMB-PII localization vector at Xba/BamH1 sites.
OscTPI full length cDNA was also cloned in pYES2 yeast expression vector (Invitrogen) at HindIII/BamH1 for yeast complementation assay.
Expression and purification of OscTPI (LOC_Os01 g62420) recombinant protein
The pET28aOscTPI construct was transformed in to BL21 (DE3) E. coli cells. The transformed cells were grown on LB medium till the OD reached about 0.5 and induced with 0.3 mM IPTG at 37°C for 4 h. OscTPI recombinant protein was purified from pellet of 500 ml bacterial culture. The pellet was dissolved and sonicated in 20 ml sonication buffer and centrifuged. The clear lysate was collected, mixed with 2 ml Ni-NTA agarose slurry by shaking gently (200 rpm on a rotary shaker) at 4°C for 60 min. The lysate–Ni-NTA mixture was loaded into a column and the protein was eluted 10 times with 1.0 ml elution buffer (50 mM sodium phosphate buffer pH 8.0, 10% glycerol, 300 mM NaCl, 1 mM PMSF, 100 mM Imidazole). The eluted fractions were analyzed by SDS-PAGE.
Determination of Specific activity and kinetic parameters of OscTPI
Specific activity of OscTPI was assayed with glyceraldehyde 3-phosphate as substrate. As previously reported,Citation59 dihydroxyacetone phosphate productions was coupled to NADH oxidation via α-glycerol phosphate dehydrogenase. Reaction mixture containing 100 mM triethanolamine hydrochloride, 10 mM EDTA, 1 mM dithiothreitol, pH 7.4, containing 0.2 mM NADH, 20 ng α-glycerol phosphate dehydrogenase and 0.1 -2 mM D-glyceraldehyde 3- phosphate was initiated by the addition of 50 ng of purified recombinant protein.The initial rate was calculated from the change in NADH absorbance at 340 nm with spectrophotometer. All experiments were performed at 25°C.
Kinetic studies for rice TPI was performed using the reaction mixture containing 0.03 mM NADH, 0.4 mM D-glyceraldehyde-3-phosphate and variable concentrations of glyceraldehyde 3-phosphate between 0.05 mM and 2.0 mM and 600 ng of purified triosephosphate isomerase in a final volume of 1 ml.
Functional complementation of yeast TPI (Tim) mutant with OscTPI
OscTPI gene was cloned in the yeast expression vector pYES2 downstream to GAL1 promoter. S. cerevisiae cells BY4741 (Mat a., his3Δ1 leu2Δ0 met15Δ0 ura3Δ0), BY4741ΔTIMI (Mat a., his3Δ1. leu2Δ0. met15Δ0. ura3Δ0. YHR057c::kanMX4) were transformed with either pYES2 alone or pYES2OscTPI and transformants were picked up by Ura prototrophy. For comparing the growth profile of these strains, cells were streaked on solid Ura- SD plate supplemented with either 20% glucose or 20% galactose and grown at 30°C and 37°C for 48 h-72 h.
Protoplast isolation and localization of TPI
Protoplast isolation and transfection for OscTPI was performed following the method of Sheen et al.Citation60 Soft and young leaves from 25 d old tobacco plants were sliced and mixed with a total of 30 ml cell wall lysis enzyme (1% cellulase Y-C, 0.1% macerozyme, (Kyowa Chemical Products, Osaka, Japan), 0.4 M mannitol, 5 mM MES, pH 5.7, filter sterilized). The mixture was incubated in a small Petridish at 25°C for 3 h in dark and rotated at (60 rpm). Protoplasts were then transferred to 2 × 50-ml corex tubes and centrifuged at low speed (145 g, Eppendorf 5810R) for 2 min. After removal of the supernatant, the protoplasts were washed thrice in 20 ml wash buffer (0.4 M mannitol, 2.5 mM CaCl2, 1 mM MES, pH 5.7) via consecutive resuspension and centrifugation. Finally, the protoplasts were resuspended in 20 ml solution containing 0.4 m mannitol, 15 mM MgCl2, 5 mM MES, pH 5.7. This procedure usually yielded 105 to 106 protoplasts per ml. After removal of the supernatant, the protoplasts were diluted in a solution containing 154 mM NaCl, 125 mM CaCl2 5.0 mM KCL, 2.0 mM MES (pH 5) and 2.0 µg of TPI-pMBGFP plasmid was added along with the 40% PEG and mixed gently. The mixture was then incubated for 20 h at 23°C with gentle agitation. The next d, incubation buffer was removed and protoplasts were viewed via confocal microscopy.
Particle bombardment and flourescence microscopy
Onion Peel Bombardment assay was performed following the method of Singh et al.Citation61 Onion epidermal peels were taken out carefully from the surface of young spring onion bulbs and placed on ½ MS-agar plates containing 1% sucrose. About 3 µg of TPI-GFP plasmid was coated with 1 µm gold particle and introduced transiently into onion epidermal cells by microprojectile bombardment, using a Bio-Rad PDS/1000 helium-driven particle accelerator, as per manufacturer's instructions. Plate was incubated at 28°C for 18 h in dark before microscopic analysis. Transformed epidermal onion peels were observed under fluorescence microscope. GFP signals were detected between 505–550 nm after exciting with 488 nm.
Native protein isolation and TPI Activity in plants
TPI activity was assayed with D-glyceraldehyde-3- phosphate and glycerophosphate dehydrogenase as a coupling enzyme followed by measuring the decrease in OD at 340 nm.Citation62 Specific activity of TPI was also measured in 12 d old rice seedlings (IR64) exposed to stress conditions. Native protein was assayed following extraction with buffer containing 0.1 M sodium phosphate buffer, pH 7.0., 50% glycerol, 16 mM MgSO4, 0.2 mM PMSF and 0.2% PVPP. Protein was quantified using Bradford method and the supernatant was immediately used for TPI assay. Two microgram of crude protein was added to the reaction mixture containing 0.03 mM NADH, 0.4 mM D-glyceraldehyde-3-phosplhate and one unit of glycerophosphate dehydrogenase. A time scan was performed spectrophotometrically, taking the OD340 at every 10 sec within a time scale that showed a linear increase in absorbance. The molar absorption coefficient of NADH at 340 nm is 6.3 × 103 m−1cm−1. The specific activity of the enzyme was expressed in µm/min/mg of protein.
Native PAGE was performed on 10% acrylamide gels and TPI activity was assayed. The gel was stained as described by Pichersky and Gottlieb.Citation13 Briefly, after native electrophoresis, gels were incubated for 20 min in equilibration buffer (0.1 M Tris–Cl pH 7.5, 0.5 mM EDTA and 0.2 mM NADH) with gentle agitation. The gel was then transferred to developing buffer containing 0.1 M Tris–Cl pH 7.5, 1 mM GAP, 0.5 mM EDTA, 0.2 mM NADH and 3 U/mL /- GPDH. When viewed over a UV transilluminator, TPI isozymes appeared within 2–5 min as dark bands over a fluorescent background. Control gels were incubated without GAP that did not show any activity band even when incubated for up to 60 min.
Cell Fractionation
Preparation of nuclear extract
Nuclear extracts were prepared according to the method described previously.Citation63 12 d old rice seedlings were homogenized and extracted using cold buffer containing 0.55 M sucrose, 50 mM Tris ± Cl pH 8.0 and 10 mM MgCl2 and the homogenate was passed through two layers of cheesecloth and two layers of Miracloth. The filtrate was then centrifuged at 1000 g for 10 min at 4°C and pellet was slowly resuspended in STM containing 2.5% TritonX100, and incubated at 4°C with slow shaking followed by centrifugation at 2000 g for 30 min at 4°C. The resulting nuclear pellet was then resuspended in a buffer containing 600 mM KCl, 50 mM Tris ± Cl pH 7.9, 1.5 mM MgCl2, 0.2 mM EDTA, 0.5 mM DTT, 25% glycerol, 0.5 mM PMSF, 50 mM leupeptin and 1 mM pepstatin, and the homogenate was spun at 12 000 g for 30 min at 4°C, and the clear supernatant (nuclear extract) was dialysed against buffer containing 50 mM KCl, 50 mM Tris ± Cl pH 8, 20% glycerol and protease inhibitors, and stored at ± 80°C.
Preparation of chloroplast lysate
Chloroplast lysate was prepared by the method earlier described earlier.Citation64 Briefly, 12 d old rice seedlings were homogenized and extracted in buffer containing 0.5 M sucrose, 50 mM Tris/HCl, pH 8.0, 10 mM MgCl,, 10 mM 2- mercaptoethanol, 0.3 mM phenylmethylsulfonyl fluoride, 1 mM sodium bisulfite and I mg/ml benzamidine . The homogenate was filtered and filtrate was centrifuged for 10 rnin at 1000 g and the pellet suspended in 120 ml of the above buffer. To disrupt the chloroplast, 30 ml 12.5% Triton X-100 was added to the chloroplast suspension. The mixture was kept on ice for 45 min, stirred occasionally and centrifuged at 3000 g for 15 min. Chloroplast lysate was used for western blot hybridization.
Western Blot Hybridization
Western blot assay was performed as described previously.Citation65 Proteins separated on SDS-PAGE were transferred to Hybond-C+ membrane (GE Healthcare) using Mini Transblot Electrophoretic cell (Biorad, USA). Electroblotting buffer consisted of 150 mM glycine, 20 mM Tris and 20% methanol (pH 8.0). The blot was incubated in blocking solution (5% non-fat dried milk dissolved in PBS) for 45 min and then washed with 0.1% PBST. Blot was further incubated with primary antibodies at specific dilution with blocking buffer for 1 h followed by incubation in Alkaline-Phosphatase conjugated secondary antibodies (diluted in blocking buffer) for 1 h. After washing, The protein-antibody complex was developed in 5-bromo-4-chloro-3-indolyl phosphate (BCIP) / nitroblue tetrazolium (NBT) containing solution (0.1 M TRIS-HCl pH 9.5, 0.1 M NaCl, 5 mM MgCl2 containing 150 µg/ml of NBT and 75 µg/ml of BCIP). The reaction was stopped by rinsing the blot with water.
Real time PCR
Real Time PCR was performed as previously standardized and described.Citation66 Total RNA was isolated from the shoot tissues of control and stressed seedlings using RaFlex™ solution I and solution II (GeNei, India), and cDNA synthesis was performed with RevertAid™ RNase H minus cDNA synthesis kit (Fermentas Life Sciences, USA). Manufacturer’s protocol was strictly followed in both the above procedures. Real time PCR primers for OscTPI were designed from 3′ UTR regions using Primer3 software. cDNA was further diluted 50 times and reaction mixture was prepared adding 5 μl of diluted cDNA, 12.5 μl of 2 × SYBR Green PCR Master Mix (Applied Biosystems, USA) and 200 nM of each gene-specific primer in a final volume of 25 μl. ABI Prism 7500 Sequence Detection System was used for qRT-PCR for 2 min a 50°C, 10 min at 95°C,and 40 cycles of 15 sec at 95°C, 1 min at 57°C, and 30 sec at 72°C in 96-well optical reaction plates (Applied Biosystems). Three technical replicates were analyzed and amplification was checked using dissociation graph, and relative expression ratio was calculated using comparative Ct value method.Citation67
Disclosure of Potential Conflicts of Interest
No potential conflicts of interest were disclosed.
References
- Miernyk JA. Glycolysis, the oxidative pentose phosphate pathway and anaerobic respiration. Plant Physiol Biochem Mol Bio 1990; 77-100.
- Banner DW, Bloomer AC, Petsko GA, Phillips DC, Pogson CI, Wilson IA, et al. Structure of chicken muscle triose phosphate isomerase determined crystallographically at 2.5 angstrom resolution using amino acid sequence data. Nature 1975; 255:609 - 14; http://dx.doi.org/10.1038/255609a0; PMID: 1134550
- Lolis E, Petsko GA. Crystallographic analysis of the complex between triosephosphate isomerase and 2-phosphoglycolate at 2.5-A resolution: implications for catalysis. Biochemistry 1990; 29:6619 - 25; http://dx.doi.org/10.1021/bi00480a010; PMID: 2204418
- Wierenga RK, Kalk KH, Hol WGJ. Structure determination of the glycosomal triosephosphate isomerase from Trypanosoma brucei brucei at 2.4 A resolution. J Mol Biol 1987; 198:109 - 21; http://dx.doi.org/10.1016/0022-2836(87)90461-X; PMID: 3430602
- Maister SG, Pett CP, Albery WJ, Knowles JR. Energetics of triosephosphate isomerase: the appearance of solvent tritium in substrate dihydroxyacetone phosphate and in product. Biochemistry 1976; 15:5607 - 12; http://dx.doi.org/10.1021/bi00670a027; PMID: 999834
- Straus D, Gilbert W. Chicken triosephosphate isomerase complements an Escherichia coli deficiency. Proc Natl Acad Sci USA 1985; 82:2014 - 8; http://dx.doi.org/10.1073/pnas.82.7.2014; PMID: 3885220
- Brown JR, Daar IO, Krug JR, Maquat LE. Characterization of the functional gene and several processed pseudogenes in the human triosephosphate isomerase gene family. Mol Cell Biol 1985; 5:1694 - 706; PMID: 4022011
- Maquat LE, Chilcote R, Ryan PM. Human triosephosphate isomerase cDNA and protein structure. Studies of triosephosphate isomerase deficiency in man. J Biol Chem 1985; 260:3748 - 53; PMID: 2579079
- Marchionni M, Gilbert W. The triosephosphate isomerase gene from maize: introns antedate the plant-animal divergence. Cell 1986; 46:133 - 41; http://dx.doi.org/10.1016/0092-8674(86)90867-6; PMID: 3755078
- Cheng J, Mielnicki LM, Pruitt SC, Maquat LE. Nucleotide sequence of murine triosephosphate isomerase cDNA. Nucleic Acids Res 1990; 18:4261; http://dx.doi.org/10.1093/nar/18.14.4261; PMID: 2377473
- McKnight GL, O’Hara PJ, Parker ML. Nucleotide sequence of the triosephosphate isomerase gene from Aspergillus nidulans: implications for a differential loss of introns. Cell 1986; 46:143 - 7; http://dx.doi.org/10.1016/0092-8674(86)90868-8; PMID: 3521890
- Sato F, Fitchen JH, Takeshita N, Hashimoto T, Okada N, Yamada Y. Synthesis of plant triosephosphate isomerase in Escherichia coli.. Agric Biol Chem 1990; 54:2189 - 91; http://dx.doi.org/10.1271/bbb1961.54.2189; PMID: 1368616
- Pichersky E, Gottlieb LD, Hess JF. Nucleotide sequence of the triose phosphate isomerase gene of Escherichia coli.. Mol Gen Genet 1984; 195:314 - 20; http://dx.doi.org/10.1007/BF00332765; PMID: 6092857
- Old SE, Mohrenweiser HW. Nucleotide sequence of the triosephosphate isomerase gene from Macaca mulatta. Nucleic Acids Res 1988; 16:9055; http://dx.doi.org/10.1093/nar/16.18.9055; PMID: 3174447
- Swinkels BW, Gibson WC, Osinga KA, Kramer R, Veeneman GH, van Boom JH, et al. Characterization of the gene for the microbody (glycosomal) triosephosphate isomerase of Trypanosoma brucei.. EMBO J 1986; 5:1291 - 8; PMID: 3015595
- Alber T, Kawasaki G. Nucleotide sequence of the triose phosphate isomerase gene of Saccharomyces cerevisiae. J Mol Appl Genet 1982; 1:419 - 34; PMID: 6759603
- Russell PR. Transcription of the triose-phosphate-isomerase gene of Schizosaccharomyces pombe initiates from a start point different from that in Saccharomyces cerevisiae.. Gene 1985; 40:125 - 30; http://dx.doi.org/10.1016/0378-1119(85)90031-9; PMID: 3912263
- Fothergill-Gilmore LA. The evolution of the glycolytic pathway. Trends Biochem Sci 1986; 11:47 - 51; http://dx.doi.org/10.1016/0968-0004(86)90233-1
- Xu MQ, Southworth MW, Mersha FB, Hornstra LJ, Perler FB. In vitro protein splicing of purified precursor and the identification of a branched intermediate. Cell 1993; 75:1371 - 7; http://dx.doi.org/10.1016/0092-8674(93)90623-X; PMID: 8269515
- Schneider AS. Triosephosphate isomerase deficiency: historical perspectives and molecular aspects. Best Pract Res Clin Haematol 2000; 13:119 - 40; http://dx.doi.org/10.1053/beha.2000.0061; PMID: 10916682
- Tanaka A, Christensen MJ, Takemoto D, Park P, Scott B. Reactive oxygen species play a role in regulating a fungus-perennial ryegrass mutualistic interaction. Plant Cell 2006; 18:1052 - 66; http://dx.doi.org/10.1105/tpc.105.039263; PMID: 16517760
- Ramasamy R, Vannucci SJ, Yan SS, Herold K, Yan SF, Schmidt AM. Advanced glycation end products and RAGE: a common thread in aging, diabetes, neurodegeneration, and inflammation. Glycobiology 2005; 15:16R - 28R; http://dx.doi.org/10.1093/glycob/cwi053; PMID: 15764591
- Thornalley PJ, Battah S, Ahmed N, Karachalias N, Agalou S, Babaei-Jadidi R, et al. Quantitative screening of advanced glycation endproducts in cellular and extracellular proteins by tandem mass spectrometry. Biochem J 2003; 375:581 - 92; http://dx.doi.org/10.1042/BJ20030763; PMID: 12885296
- Ahmed N, Battah S, Karachalias N, Babaei-Jadidi R, Horányi M, Baróti K, et al. Increased formation of methylglyoxal and protein glycation, oxidation and nitrosation in triosephosphate isomerase deficiency. Biochim Biophys Acta 2003; 1639:121 - 32; http://dx.doi.org/10.1016/j.bbadis.2003.08.002; PMID: 14559119
- Inoue Y, Kimura A. Methylglyoxal and regulation of its metabolism in microorganisms. Adv Microb Physiol 1995; 37:177 - 227; http://dx.doi.org/10.1016/S0065-2911(08)60146-0; PMID: 8540421
- Yadav SK, Singla-Pareek SL, Ray M, Reddy MK, Sopory SK. Methylglyoxal levels in plants under salinity stress are dependent on glyoxalase I and glutathione. Biochem Biophys Res Commun 2005; 337:61 - 7; http://dx.doi.org/10.1016/j.bbrc.2005.08.263; PMID: 16176800
- Ting SM, Miller ON, Sellinger OZ. The metabolism of lactaldehyde with the oxidation of d-lactaldehyde in rat liver. Biochim Biophys Acta 1965; 97:407 - 15; http://dx.doi.org/10.1016/0304-4165(65)90151-0; PMID: 14323585
- Corran PH, Waley SG. The amino acid sequence of rabbit muscle triosephosphate isomerase. Biochem J 1990; 145:335 - 44
- Artavanis-Tsakonas S, Harris JI. Primary structure of triosephosphate isomerase from Bacillus stearothermophilus.. Eur J Biochem 1980; 108:599 - 611; http://dx.doi.org/10.1111/j.1432-1033.1980.tb04755.x; PMID: 6105959
- Tomlison JD, Turner JF. Pea seed triosephosphate isomerase. Phytochemistry 1979; 18:1959 - 62; http://dx.doi.org/10.1016/S0031-9422(00)82711-9
- Bukowiecki C, Anderson LE. Multiple forms of aldolase and triosephosphate isomerase in diverse plant species. Plant Sci Lett 1974; 3:381 - 6; http://dx.doi.org/10.1016/0304-4211(74)90019-4
- Chen M, Thelen JJ. The plastid isoform of triose phosphate isomerase is required for the postgerminative transition from heterotrophic to autotrophic growth in Arabidopsis. Plant Cell 2010; 22:77 - 90; http://dx.doi.org/10.1105/tpc.109.071837; PMID: 20097871
- Garza-Ramos G, Pérez-Montfort R, Rojo-Domínguez A, de Gómez-Puyou MT, Gómez-Puyou A. Species-specific inhibition of homologous enzymes by modification of nonconserved amino acids residues. The cysteine residues of triosephosphate isomerase. Eur J Biochem 1996; 241:114 - 20; http://dx.doi.org/10.1111/j.1432-1033.1996.0114t.x; PMID: 8898895
- Dorion S. Parveen, Jeukens J, Matton DP, Rivoal J. Cloning and characterization of a cytosolic isoform of triosephosphate isomerase developmentally regulated in potato leaves. Plant Sci 2005; 168:183 - 94; http://dx.doi.org/10.1016/j.plantsci.2004.07.029
- Henze K, Schnarrenberger C, Kellermann J, Martin W. Chloroplast and cytosolic triosephosphate isomerases from spinach: purification, microsequencing and cDNA cloning of the chloroplast enzyme. Plant Mol Biol 1994; 26:1961 - 73; http://dx.doi.org/10.1007/BF00019506; PMID: 7858230
- Gracy RW. Triosephosphate isomerase from human erythrocytes. Methods Enzymol 1975; 41:442 - 7; http://dx.doi.org/10.1016/S0076-6879(75)41096-5; PMID: 1128275
- Repiso A, Boren J, Ortega F. Triosephosphateisomerase deficiency: Genetic, enzymatic and metabolic characterization of a new case from Spain. Haematologica 2002; 87:804 - 12; PMID: 12161355
- Krietsch WKG, Bücher T. 3-phosphoglycerate kinase from rabbit sceletal muscle and yeast. Eur J Biochem 1970; 17:568 - 80; http://dx.doi.org/10.1111/j.1432-1033.1970.tb01202.x; PMID: 5493986
- Hartman FC, Ratrie H 3rd. Apparent equivalence of the active-site glutamyl residue and the essential group with pKalpha 6.0 in triosephosphate isomerase. Biochem Biophys Res Commun 1977; 77:746 - 52; http://dx.doi.org/10.1016/S0006-291X(77)80041-7; PMID: 20092
- Lambeir AM, Opperdoes FR, Wierenga RK. Kinetic properties of triose-phosphate isomerase from Trypanosoma brucei brucei. A comparison with the rabbit muscle and yeast enzymes. Eur J Biochem 1987; 168:69 - 74; http://dx.doi.org/10.1111/j.1432-1033.1987.tb13388.x; PMID: 3311744
- Go MK, Koudelka A, Amyes TL, Richard JP. Role of Lys-12 in catalysis by triosephosphate isomerase: a two-part substrate approach. Biochemistry 2010; 49:5377 - 89; http://dx.doi.org/10.1021/bi100538b; PMID: 20481463
- Albery WJ, Knowles JR. Free-energy profile of the reaction catalyzed by triosephosphate isomerase. Biochemistry 1976; 15:5627 - 31; http://dx.doi.org/10.1021/bi00670a031; PMID: 999838
- Weeden NF, Gottlieb LD. Isolation of cytoplasmic enzymes from pollen. Plant Physiol 1980; 66:400 - 3; http://dx.doi.org/10.1104/pp.66.3.400; PMID: 16661444
- Anderson LE. Chloroplast and cytoplasmic enzymes. II. Pea leaf triose phosphate isomerases. Biochim Biophys Acta 1971; 235:237 - 44; http://dx.doi.org/10.1016/0005-2744(71)90051-9; PMID: 5089710
- Umeda M, Uchimiya H. Differential transcript levels of genes associated with glycolysis and alcohol fermentation in rice plants under submergence stress. Plant Physiol 1994; 106:1015 - 22; PMID: 12232382
- Kawasaki S, Borchert C, Deyholos M, Wang H, Brazille S, Kawai K, et al. Gene expression profiles during the initial phase of salt stress in rice. Plant Cell 2001; 13:889 - 905; PMID: 11283343
- Mustroph A, Albrecht G. Tolerance of crop plants to oxygen deficiency stress: fermentative activity and photosynthetic capacity of entire seedlings under hypoxia and anoxia. Physiol Plant 2003; 117:508 - 20; http://dx.doi.org/10.1034/j.1399-3054.2003.00051.x; PMID: 12675741
- Riccardi F, Gazeau P, Zivy M, Zivy M, de Vienne D. Protein changes in response to progressive water deficit in maize. Quantitative variation and polypeptide identification. Plant Physiol 1998; 117:1253 - 63; http://dx.doi.org/10.1104/pp.117.4.1253; PMID: 9701581
- Hernández P, Dorado G, Laurie DA, Martin A, Snape JW. Microsatellites and RFLP probes from maize are efficient sources of molecular markers for the biomass energy crop Miscanthus. Theor Appl Genet 2001; 102:616 - 22; http://dx.doi.org/10.1007/s001220051688
- Inose T, Murata K. Enhanced accumulation of toxic compound in yeast cells having high glycolytic activity: a case study on the safety of genetically engineered yeast. Int J Food Sci Technol 1995; 30:141 - 6; http://dx.doi.org/10.1111/j.1365-2621.1995.tb01365.x
- Di Loreto S, Caracciolo V, Colafarina S, Sebastiani P, Gasbarri A, Amicarelli F. Methylglyoxal induces oxidative stress-dependent cell injury and up-regulation of interleukin-1β and nerve growth factor in cultured hippocampal neuronal cells. Brain Res 2004; 1006:157 - 67; http://dx.doi.org/10.1016/j.brainres.2004.01.066; PMID: 15051519
- Chang KC, Paek KS, Kim HJ, Lee YS, Yabe-Nishimura C, Seo HG. Substrate-induced up-regulation of aldose reductase by methylglyoxal, a reactive oxoaldehyde elevated in diabetes. Mol Pharmacol 2002; 61:1184 - 91; http://dx.doi.org/10.1124/mol.61.5.1184; PMID: 11961137
- Chen G, Gharib TG, Huang CC, Taylor JM, Misek DE, Kardia SL, et al. Discordant protein and mRNA expression in lung adenocarcinomas. Mol Cell Proteomics 2002; 1:304 - 13; http://dx.doi.org/10.1074/mcp.M200008-MCP200; PMID: 12096112
- Hajduch M, Hearne LB, Miernyk JA, Casteel JE, Joshi T, Agrawal GK, et al. Systems analysis of seed filling in Arabidopsis: using general linear modeling to assess concordance of transcript and protein expression. Plant Physiol 2010; 152:2078 - 87
- Mankikar S, Rangekar P. Effects of methylglyoxal on germination of barley. Fyton 1974; 32:9 - 16
- Samanez-Larkin GR, Gibbs SE, Khanna K, Nielsen L, Carstensen LL, Knutson B. Anticipation of monetary gain but not loss in healthy older adults. Nat Neurosci 2007; 10:787 - 91; http://dx.doi.org/10.1038/nn1894; PMID: 17468751
- Itoh J, Nonomura K, Ikeda K, Yamaki S, Inukai Y, Yamagishi H, et al. Rice plant development: from zygote to spikelet. Plant Cell Physiol 2005; 46:23 - 47; http://dx.doi.org/10.1093/pcp/pci501; PMID: 15659435
- Yoshida S, Forno DA, Cock JH, Gomez KA. Laboratory manual for physiological studies of rice. International Rice Research Institute 1972, Manila.
- Rozacky EE, Sawyer TH, Barton RA, Gracy RW. Studies on human triosephosphate isomerase. I. Isolation and properties of the enzyme from erythrocytes. Arch Biochem Biophys 1971; 146:312 - 20; http://dx.doi.org/10.1016/S0003-9861(71)80069-3; PMID: 5169140
- Sheen J. Signal transduction in maize and Arabidopsis mesophyll protoplasts. Plant Physiol 2001; 127:1466 - 75; http://dx.doi.org/10.1104/pp.010820; PMID: 11743090
- Singh A, Baranwal V, Shankar A, Kanwar P, Ranjan R, Yadav S, et al. Rice phospholipase A superfamily: organization, phylogenetic and expression analysis during abiotic stresses and development. PLoS ONE 2012; 7:e30947; http://dx.doi.org/10.1371/journal.pone.0030947; PMID: 22363522
- Maitra PK, Lobo Z. A kinetic study of glycolytic enzyme synthesis in yeast. J Biol Chem 1971; 246:475 - 88; PMID: 5542016
- Tuteja N, Beven AF, Shaw PJ, Tuteja R. A pea homologue of human DNA helicase I is localized within the dense fibrillar component of the nucleolus and stimulated by phosphorylation with CK2 and cdc2 protein kinases. Plant J 2001; 25:9 - 17; http://dx.doi.org/10.1046/j.1365-313x.2001.00918.x; PMID: 11169178
- Tuteja N, Phan TN, Tewari KK. Purification and characterization of a DNA helicase from pea chloroplast that translocates in the 3′-to-5′ direction. Eur J Biochem 1996; 238:54 - 63; http://dx.doi.org/10.1111/j.1432-1033.1996.0054q.x; PMID: 8665952
- Burnette WN. “Western blotting”: electrophoretic transfer of proteins from sodium dodecyl sulfate--polyacrylamide gels to unmodified nitrocellulose and radiographic detection with antibody and radioiodinated protein A. Anal Biochem 1981; 112:195 - 203; http://dx.doi.org/10.1016/0003-2697(81)90281-5; PMID: 6266278
- Mustafiz A, Singh AK, Pareek A, Sopory SK, Singla-Pareek SL. Genome-wide analysis of rice and Arabidopsis identifies two glyoxalase genes that are highly expressed in abiotic stresses. Funct Integr Genomics 2011; 11:293 - 305; http://dx.doi.org/10.1007/s10142-010-0203-2; PMID: 21213008
- Livak KJ, Schmittgen TD. Analysis of relative gene expression data using real-time quantitative PCR and the 2(-Delta Delta C(T)). Method. Methods 2001; 25:402 - 8; http://dx.doi.org/10.1006/meth.2001.1262; PMID: 11846609