Abstract
The function of hormones during plant growth, development and response to environmental stresses relies heavily upon the actions of the ubiquitin proteasome system (UPS), which selectively degrades numerous proteins. Synthesis of ethylene, a growth and stress hormone, is regulated in part by the ubiquitin-dependent degradation of the rate-limiting enzymatic protein aminocyclopropane-1-carboxylic acid synthase (ACS). Regulation of ACS protein stability, and therefore ethylene production, is mediated by non-catalytic sequences within the C-terminal extension of many ACS proteins. In this review we provide a brief overview of the E3 ligases that target ACS proteins for degradation and discuss how post-translational modification of the C-terminal extensions influence protein stability.
The selective degradation of proteins through the ubiquitin 26S proteasome system (UPS) is required for many cellular processes and subsequently has a major impact on plant physiology. The UPS mediates responses to both abiotic and biotic stress and it facilitates normal growth and development of plants. In many cases, the UPS functions to regulate hormone biosynthesis, perception and/or signal transduction. Similar to ABA, auxin and gibberellins, ethylene biosynthesis and signal transduction is heavily regulated through ubiquitin-dependent degradation.Citation1 Across plant species, members of the ethylene biosynthetic gene family, aminocyclopropane-1-carboxylic acid synthase (ACS), have been shown to be subject to ubiquitin-dependent degradation.Citation2 ACS proteins catalyze the rate limiting step in ethylene biosynthesis; the conversion of S-adenosylmethionine (AdoMet) to 1-aminocyclopropane-1-carboxylic acid (ACC).Citation3,Citation4 Members of the ACS family share a high degree of sequence similarity in their catalytic domain and contain variable C-terminal extensions.Citation5 The ACS C-terminal sequences contribute to the stability of the ACS enzymes. In this review we discuss the regulation of ACS proteins through ubiquitin-dependent degradation.
Ubiquitination
Ubiquitin is covalently attached to target proteins through the sequential actions of three enzymes; E1 (ubiquitin activating enzyme), E2 (ubiquitin conjugating enzyme) and E3 (ubiquitin ligase). The conjugation process begins with the ATP-dependent activation of ubiquitin by the E1 enzymes. Following activation, the ubiquitin molecule is transferred to the E2 forming a thioester linked E2-ubiquitin intermediate. The E3 binds to both the E2-ubquitin intermediate and the target protein. Both the E2 and E3 enzymes coordinate the transfer of ubiquitin onto a lysine residue of the selected target. This process can be repeated creating a chain of ubiquitin molecules on the target. The outcome of ubiquitination varies depending upon the number of ubiquitin molecules and the topology of the ubiquitin chain attached to the target. Ubiquitination can influence protein function by directing cellular localization, modulating activation and controlling abundance.Citation6,Citation7 The attachment of a ubiquitin chain formed using lysine 48 linkages marks the target protein for degradation by the 26S proteasome.
Ubiquitin ligases determine substrate specificity of the ubiquitination process. This important role is reflected in the large number of E3 ligases encoded by plant genomes compared with the smaller number of E1 and E2s. For example, Arabidopsis has only two genes that encode for E1s, 37 genes that encode for E2s and over 1,300 genes that encode for E3 ligases.Citation8,Citation9 Ubiquitin ligases can be categorized into three broad groups, HECT-, U box- or RING-type, based on the domain used to interact with the E2-ubiquitin intermediate. RING- and U box-type E3s function by facilitating the transfer of ubiquitin directly from the E2 to the target protein. The HECT-type E3s are unique because they form a E3-ubiquitin intermediate prior to transfer of the ubiquitin to the target protein.Citation10 RING-type E3 ligases function as monomeric enzymes, which contain both the E2 and substrate binding domain on the same polypeptide, or as multi-subunit E3s with the E2 and substrate binding functions found on separate proteins. The multi-subunit E3 ligases contain a cullin (CUL) protein, an E2 binding RING Box1 (RBX1a/b) protein and a substrate binding protein.Citation11 The broad complex/tramtrack/bric-a-brac (BTB) proteins are examples of a substrate recruiting proteins that form an E3 ligase complex with CUL3a/b.Citation12 Some cullin-based E3 ligases require an additional adaptor protein to mediate interaction between the CUL protein and the substrate binding protein. For example, CUL1 uses the adaptor protein S-Phase Kinase-associated Protein (SKP) to bind to the substrate-recruiting F-box proteins.Citation13
Both E3 ligases and targets of ubiquitination are regulated such that degradation only occurs under specific conditions. During most stages of plant development ethylene production is kept low in part through ubiquitination and degradation of ACS proteins.Citation2,Citation14 In response to specific developmental or environmental cues, such as wounding or pathogen attack, the ubiquitin-dependent degradation of specific ACS proteins is slowed or prevented such that ACS proteins can accumulate and contribute to a burst of ethylene production. The resulting ethylene mediates relevant changes in plant physiology.
The factors that influence of the stability of ACS proteins from Arabidopsis, tomato (Lycopersicon esculentum) and other species have been widely studied. It appears that while the catalytic domain of these proteins is highly conserved, the C-terminal extensions are highly variable and facilitate the specific degradation patterns of the different ACS isoforms. Arabidopsis ACS proteins fall into three groups depending on the presence of phosphorylation sites in their C-terminal extension ().Citation2 Type 1 ACS proteins (ACS1, 2 and 6) have the longest extensions and contain three mitogen-activated protein kinase (MAPK) phosphorylation sites and one calcium‐dependent protein kinase (CDPK) phosphorylation site. Type 2 ACS proteins (ACS4, 5, 8, 9 and 11) contain a CDPK phosphorylation site embedded within a specific sequence (referred to as a Target Of ETO1 or TOE) required for interaction with ethylene-overproducer1 (ETO1).Citation15 Type 3 ACS proteins are interesting because they have a truncated C-terminal extension containing neither phosphorylation sites or TOE sequence. There is only one type 3 ACS protein in Arabidopsis (ACS7) but type 3 ACS proteins are common among many plant species including cucumber (Cucumis sativus) and poplar (Populus trichocarpa).Citation16
Figure 1. A. Classification of Arabidopsis and tomato (Lycopersicon esculentum) ACS proteins based on phosphorylation sites within the C-terminal extension. Type 1 ACS proteins contain one CDPK and three MAPK phosphorylation sites. Type 2 ACS proteins contain one CDPK phosphorylation site and a target of ETO1 (TOE) sequence. Type 3 ACS proteins contain neither phosphorylation sites nor TOE sequence. B. Factors that influence the stability of Arabidopsis ACS proteins. Type 1 ACS protein are phosphorylated by MPK3/6 in response to external stimuli such as Botrytis cinerea infection and are possibly phosphorylated by CDPKs. Phosphorylated type 1 ACS proteins are more stable than unphosphorylated forms. Dephosphorylation by PP2A renders type 1 ACS proteins unstable. Type 2 ACS proteins are ubiquitinated by ETO1 and EOL1/2 ubiquitin ligases and are stabilized by cytokinin or brassinosteroid treatment. It is unclear how CDPK phosphorylation affects protein stability. The type 3 ACS protein is ubiquitinated and targeted for degradation by the E3 ligase XBAT32. Factors that influence the stability of type 3 ACS proteins are unknown. P, phosphate group; S, serine residue
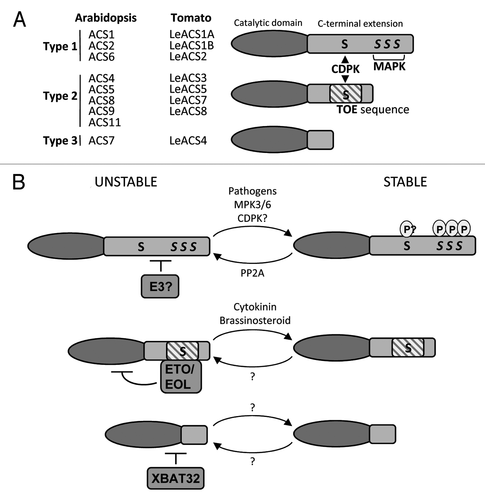
E3 Ligases that Target ACS Proteins
The stability of type 2 and type 3 ACS proteins is dependent upon the activity of RING-type E3 ligases (). ETO1 is a BTB protein that functions as the substrate recruiting component of a CUL3a/b based E3 ligase complex.Citation12,Citation17 The eto1 loss of function plants overproduce ethylene and dark-grown seedlings display a constitutive triple response phenotype which can be partially rescued by loss of ACS5.Citation18,Citation19 ETO1-like1 and ETO1-like2 (EOL1 and EOL2) are homologous to ETO1 and all three are thought to contribute to the ubiquitin-dependent degradation of the type 2 ACS proteins.Citation20 ETO1, EOL1 and EOL2 bind to the type 2 ACS proteins through the C-terminal TOE sequence.Citation15 eto2 and eto3 are dominant negative mutations in the TOE sequence of ACS5 and ACS9, respectively, which interrupts binding to ETO1/EOL1 and reduces degradation of the ACSs. The resulting accumulation of either ACS5 eto2 or ACS9eto3 accounts for the overproduction of ethylene observed in eto mutants.Citation18,Citation19
Ethylene production can be induced in Arabidopsis by treatment with cytokinin and brassinosteroid hormones.Citation18,Citation21 Both hormones appear to induce ethylene biosynthesis through stabilization of ACS5 and ACS9.Citation18,Citation21 The mechanism by which ACS5 and ACS9 are protected from degradation is unclear because both ACS5eto2 and ACS9eto3 mutations, which have compromised binding to ETO1/EOL1, are further stabilized by cytokinin and brassinosteroid. It is suggested that ACS5 and ACS9 binding to ETO1/EOL1 is only partially disrupted by the eto2 and eto3 mutations and that cytokinin and brassinosteroid further prohibits this interaction.Citation18,Citation21 Another possibility is that additional E3s contribute to the degradation of type 2 ACS proteins and cytokinin and brassinosteroid affect the activity of these ubiquitin ligases. The Arabidopsis type 3 ACS (ACS7) does not interact with ETO1 or EOL1.Citation20 Similarly, a type 3 ACS protein from tomato (LeACS4) does not interact with the ETO1.Citation22 This suggests that type 3 ACSs are not regulated through ETO1-dependent degradation and may be regulated through a different mechanism.
XBAT32 is a monomeric RING-type E3 ligase involved in ethylene homeostasis. Recently, XBAT32 was shown to be required for the degradation of ACS4 and ACS7.Citation16 XBAT32 mutants overproduces ethylene and displays a number of ethylene-related phenotypes including a mild triple response in dark-grown seedlings, reduced plant height and a decrease in the number of lateral roots. XBAT32 interacts with and ubiquitinates ACS4 in vitro.Citation23 The half-life of ACS4 is extended in xbat32 plants compared with wild type suggesting that, in addition to ETO1 and EOL1/2, XBAT32 also contributes to the ubiquitin-dependent turnover of ACS4.Citation16 The role of XBAT32 in regulating the abundance of type 2 ACS protein may be limited because the E3 does not interact with ACS11.Citation23 However, its ability to interact with or affect the stability of ACS5 or ACS9 has not yet been addressed.
Type 3 ACS proteins lack known points of regulation in their C-terminal extension (). Whether or not these proteins are regulated through ubiquitin-dependent degradation was unclear. It was suggested that regulation of ACS7 abundance occurs mainly through transcriptional control since external stimuli, such as Botytis cinerea infection, induce expression of ACS7.Citation24 In our recent paper we demonstrated that ACS7 is turned over in a proteasome-dependent manner.Citation16 XBAT32 can interact with and ubiquitinate ACS7 in vitro.Citation22 The stability of ACS7 has been examined in planta in both xbat32 and wild type plants. While ACS7 is turned over in wild type plants, protein levels are stable in the xbat32 plants.Citation16 Thus, despite the lack of a C-terminal extension, type 3 ACS is regulated by the UPS.
Phosphorylation and ACS Protein Stability
Post-translational modifications through the addition of phosphate groups can influence protein activity by inducing changes in conformation, promoting specific protein-protein interactions and modulating abundance. Much of our understanding of the effect that phosphorylation has the on the stability of ACS proteins has been revealed by studying type 1 ACS proteins. A variety of evidence points to a model in which unphosphorylated type 1 ACS are rapidly degraded by the 26S proteasome under normal growth conditions thereby maintaining low ethylene levels ().Citation14 However, under certain conditions such as wounding or pathogen attack the type 1 ACS proteins are phosphorylated, become stabilized, and contribute to increased ethylene biosynthesis.Citation2,Citation14,Citation25-Citation28 Evidence to support this model comes from the observation that transgenic plants expressing the ACS6 gene under the control of the constitutively active 35S promoter does not overly produce ethylene.Citation25,Citation26 This is due to the turnover of ACS6 by the 26S proteasome. The C-terminal extension of ACS6 is enough to target a GFP-reporter protein for proteasome-dependent degradation.Citation26 Mutations of the three MAPK phosphorylation sites on the C-terminal extension of ACS6 to phosphomimic mutation (Serine to Aspartic acid, ACS6DDD) rendered ACS6 stable and resulted in ethylene related phenotypes.Citation25,Citation26 Transgenic plants overexpressing ACS6DDD displayed hairy roots, inhibition of primary root length and dwarfism.
Similar results have also been found in other species including tomato (Lycopersicon esculentum, Le), banana (Musa acuminate, Ma) and cotton (Gossypium hirsutum, Gh).Citation27,Citation29-Citation30 Treatment with kinase inhibitors decreased the phosphorylation and abundance of tomato ACS2 (LeACS2), while the phosphatase inhibitor okadaic acid produced the opposite effect.Citation27 Interestingly, both a MAP Kinase kinase (MAPKK) inhibitor (U0126) and a CDPK inhibitor (CMZ) were able to reduce the accumulation of LeACS2. This suggests that phosphorylation by both MAPKs and CDPKs contribute to the stabilization of type 1 ACS proteins. The stability of banana ACS1 (MA-ACS1), which is induced in response to ripening signals, appears to also be regulated through phosphorylation. Kinase inhibitor treatments decreased the level of phosphorylated MA-ACS1 and decreased the relative protein abundance.Citation29 Similar to type 1 ACS proteins from Arabidopsis and tomato, MA-ACS1 is phosphorylated in the C-terminal extension and a MAPKKK-like protein kinase (MA-MAPKKK) may be responsible for this phosphorylation. The cotton CDPK, GhCPK1, was also found to phosphorylate GhACS2 and phosphorylated GhACS2 may contribute to elevated ethylene biosynthesis during fast fiber elongation.Citation30
The addition of phosphate groups to the C-terminal extension increases the negative charge of the extension and may promote or prevent the binding of additional proteins. The C-terminal extension of ACS6, and other type 1 ACS proteins, contains a pair of aspartic acids which contribute to the overall charge of the extension. Interestingly, mutation of these aspartic acids to positively charged arginines reversed the stability of ACS6DDD. While ACS6DDD is stable, ACS6DDD with the pair of aspartic acids mutated to arginines (ACS6RR-DDD) is unstable.Citation26 The C-terminal extension of ACS6 also contains two clusters of positively charged lysines. Increasing the negative charge of the extension due to the mutations of these lysines to aspartic acid, was enough to stabilize ACS6 without the need for phosphomimic mutations at the MAPK sites.Citation26 The overall charge of the C-terminal extension of ACS7 and ACS4 also appears to influence their degradation rate. A mutation of the only lysine in the C-terminal extension of ACS7 to arginine, a modest increase in positive charge, was enough to increase the degradation rate of ACS7.Citation16 Similarity, mutations of four lysines on the C-terminal extension of ACS4 to arginines also increased its degradation rate.Citation16 It appears that the stability of ACS proteins is commonly influenced by the C-terminal extension. These results suggest a model whereby the C-terminal extension of type 1 ACS proteins is a key point of regulation and a more negatively charge extension is stable whereas a more positively charged extension in unstable.
The role of the single CDPK phosphorylation site found on type 2 ACS proteins is still under investigation. ACS5 proteins containing CDPK phosphomimic or phospho-null mutations are still able to interact with ETO1 or EOL1.Citation20 However, the valine to aspartic acid mutation found on ACS9eto3, may mimic phosphorylation.Citation18 ACS9eto3 is more stable that ACS9, but it is not known if the mutation represents a relevant physiological change on ACS9 that prevents binding to ETO1 or EOL1 under specific conditions. There is some evidence which suggests that phosphorylation may play a more complicated role in the stability of type 2 ACS proteins. Unlike type 1 ACS proteins, ACS5 is not stabilized by phosphatase inhibitors.Citation31 ACS5 was actually more unstable following treatment with the protein phosphatase inhibitor cantharidin. By inhibiting phosphatase activities, cantharidin may be increasing the phosphorylation status of ACS5 which results in increased degradation. Alternatively, cantharidin may influence the phosphorylation of other proteins that affect ACS5 such as ETO1, EOL1 and EOL2 and thereby indirectly influencing the turnover of ACS5.
While type 3 ACS proteins lack these phosphorylation sites, there is some indirect evidence that ACS7 is phosphorylated. ACS7 was found to interact with 14–3-3 proteins which usually require a phosphorylated recognition motif for interaction.Citation32 It is possible that ACS7 is phosphorylated somewhere within the catalytic domain. The effect of kinase or phosphatase inhibitors on the stability of ACS7 has not been addressed. Another possibility is that regulation of its corresponding E3 ligase, XBAT32, contributes to the modulation of ACS7 protein stability.
Kinases and Phosphatases that Regulate ACS Protein Stability
The three tiered MAP kinase cascade is activated in response to various stimuli and elicits a relevant physiological response including an increase in ethylene production. Tobacco and Arabidopsis MAPKs, SIPK/WIPK and MPK6/3, are activated under a variety of conditions that induce ethylene production including infection by the fungi B. cinerea.Citation24,Citation28,Citation33-Citation35 Activated MPK6 and MPK3 can phosphorylate ACS6 and ACS2 in vitro.Citation28 In a conditionally rescued mpk3/mpk6 double mutant the level of ACS6 activity is severely reduced in response to infection.Citation28 Correspondingly, the MPK3/6 phosphorylation sites on ACS6 are also required for induction of ACS6 activity in response to infection. These results are consistent with a model in which B. cinerea and/or other environmental conditions induce ethylene production through activation of MPK3/6 and subsequent phosphorylation of ACS2 and ACS6 leading to enzyme stabilization and subsequent increased ethylene production.Citation28
In opposition to the effect of MPK3/6 phosphorylation, it appears that protein phosphatase 2A interacts with ACS6 and dephosphorylates the C-terminal extension.Citation31 ACS6 is stabilized in the rcn1–6 line, which lacks a protein phosphatase 2A (PP2A) subunit and has reduced phosphatase activity. The stabilization of ACS6 in rcn1–6, due to reduced dephosphorylation of the enzyme, may contribute to the ethylene over-production observed in the RCN1 mutants.Citation31,Citation36
Conclusions
The UPS plays significant roles in the regulation of ethylene biosynthesis. The three categories of ACS proteins, as defined by their C-terminal extension, reflects different mechanisms of regulation. Despite these differences, the abundance and hence activity of the enzymes from all three categories are regulated by the UPS. The role of phosphorylation in determining the stability of type 1 ACS proteins has been somewhat defined, however the E3 ligase(s) responsible for ubiquitination remain unknown. E3 ligases that target type 2 and type 3 ACS have been identified but the mechanisms that modulate stability under different physiological conditions remains poorly understood. Continued research on the turnover of ACS proteins will provide further insights into ethylene production and its role in plant growth, development and response to environmental stresses.
Abbreviations: | ||
UPS | = | ubiquitin proteasome system |
ACS | = | aminocyclopropane-1-carboxylic acid synthase |
AdoMet | = | S-adenosylmethionine |
CUL | = | Cullin |
RING | = | Really interesting new gene |
RBX1a/b | = | RING Box1a/b |
XBAT32 | = | XB3 ortholog 2 in Arabidopsis thaliana |
BTB | = | broad complex/tramtrack/bric-a-brac |
SKP | = | S-Phase Kinase-associated Protein |
MAPK | = | mitogen-activated protein kinase |
CDPK | = | calcium‐dependent protein kinase |
ETO1 | = | Ethylene-overproducer1 |
TOE | = | target of ETO1 |
EOL1/2 | = | ETO1-like1 and ETO1-like2 |
Acknowledgments
The authors would like to thank Lucy Federico for comments on the manuscript. SLS is supported by grants the Natural Sciences and Engineering Research Council of Canada (NSERC). WJL is supported by a post-graduate scholarship from NSERC.
References
- Santner A, Estelle M. The ubiquitin-proteasome system regulates plant hormone signaling. Plant J 2010; 61:1029 - 40; 10.1111/j.1365-313X.2010.04112.x; PMID: 20409276
- Argueso CT, Hansen M, Kieber JJ. Regulation of ethylene biosynthesis. J Plant growth 2007; 26:92–105.
- Yang SF, Hoffman NE. Ethylene biosynthesis and its regulation in higher plants. Annu Rev Plant Physiol 1984; 35:155 - 89; 10.1146/annurev.pp.35.060184.001103
- Wang KL, Li H, Ecker JR. Ethylene biosynthesis and signaling networks. Plant Cell 2002; 14:Suppl S131 - 51; PMID: 12045274
- Yamagami T, Tsuchisaka A, Yamada K, Haddon WF, Harden LA, Theologis A. Biochemical diversity among the 1-amino-cyclopropane-1-carboxylate synthase isozymes encoded by the Arabidopsis gene family. J Biol Chem 2003; 278:49102 - 12; 10.1074/jbc.M308297200; PMID: 12968022
- Mukhopadhyay D, Riezman H. Proteasome-independent functions of ubiquitin in endocytosis and signaling. Science 2007; 315:201 - 5; 10.1126/science.1127085; PMID: 17218518
- Smalle J, Vierstra RD. The ubiquitin 26S proteasome proteolytic pathway. Annu Rev Plant Biol 2004; 55:555 - 90; 10.1146/annurev.arplant.55.031903.141801; PMID: 15377232
- Stone SL, Hauksdóttir H, Troy A, Herschleb J, Kraft E, Callis J. Functional analysis of the RING-type ubiquitin ligase family of Arabidopsis. Plant Physiol 2005; 137:13 - 30; 10.1104/pp.104.052423; PMID: 15644464
- Vierstra RD. The ubiquitin-26S proteasome system at the nexus of plant biology. Nat Rev Mol Cell Biol 2009; 10:385 - 97; 10.1038/nrm2688; PMID: 19424292
- Scheffner M, Nuber U, Huibregtse JM. Protein ubiquitination involving an E1-E2-E3 enzyme ubiquitin thioester cascade. Nature 1995; 373:81 - 3; 10.1038/373081a0; PMID: 7800044
- Schwechheimer C, Calderon Villalobos LI. Cullin-containing E3 ubiquitin ligases in plant development. Curr Opin Plant Biol 2004; 7:677 - 86; 10.1016/j.pbi.2004.09.009; PMID: 15491916
- Gingerich DJ, Gagne JM, Salter DW, Hellmann H, Estelle M, Ma L, et al. Cullins 3a and 3b assemble with members of the broad complex/tramtrack/bric-a-brac (BTB) protein family to form essential ubiquitin-protein ligases (E3s) in Arabidopsis. J Biol Chem 2005; 280:18810 - 21; 10.1074/jbc.M413247200; PMID: 15749712
- Lechner E, Achard P, Vansiri A, Potuschak T, Genschik P. F-box proteins everywhere. Curr Opin Plant Biol 2006; 9:631 - 8; 10.1016/j.pbi.2006.09.003; PMID: 17005440
- Chae HS, Kieber JJ. Eto Brute? Role of ACS turnover in regulating ethylene biosynthesis. Trends Plant Sci 2005; 10:291 - 6; 10.1016/j.tplants.2005.04.006; PMID: 15949763
- Yoshida H, Wang KL, Chang CM, Mori K, Uchida E, Ecker JR. The ACC synthase TOE sequence is required for interaction with ETO1 family proteins and destabilization of target proteins. Plant Mol Biol 2006; 62:427 - 37; 10.1007/s11103-006-9029-7; PMID: 16897471
- Lyzenga WJ, Booth JK, Stone SL. The Arabidopsis RING-type E3 ligase XBAT32 mediates the proteasomal degradation of the ethylene biosynthetic enzyme, 1-aminocyclopropane-1-carboxylate synthase 7. Plant J 2012; 71:23 - 34; 10.1111/j.1365-313X.2012.04965.x; PMID: 22339729
- Wang KL, Yoshida H, Lurin C, Ecker JR. Regulation of ethylene gas biosynthesis by the Arabidopsis ETO1 protein. Nature 2004; 428:945 - 50; 10.1038/nature02516; PMID: 15118728
- Chae HS, Faure F, Kieber JJ. The eto1, eto2, and eto3 mutations and cytokinin treatment increase ethylene biosynthesis in Arabidopsis by increasing the stability of ACS protein. Plant Cell 2003; 15:545 - 59; 10.1105/tpc.006882; PMID: 12566591
- Vogel JP, Woeste KE, Theologis A, Kieber JJ. Recessive and dominant mutations in the ethylene biosynthetic gene ACS5 of Arabidopsis confer cytokinin insensitivity and ethylene overproduction, respectively. Proc Natl Acad Sci U S A 1998; 95:4766 - 71; 10.1073/pnas.95.8.4766; PMID: 9539813
- Christians MJ, Gingerich DJ, Hansen M, Binder BM, Kieber JJ, Vierstra RD. The BTB ubiquitin ligases ETO1, EOL1 and EOL2 act collectively to regulate ethylene biosynthesis in Arabidopsis by controlling type-2 ACC synthase levels. Plant J 2009; 57:332 - 45; 10.1111/j.1365-313X.2008.03693.x; PMID: 18808454
- Hansen M, Chae HS, Kieber JJ. Regulation of ACS protein stability by cytokinin and brassinosteroid. Plant J 2009; 57:606 - 14; 10.1111/j.1365-313X.2008.03711.x; PMID: 18980656
- Yoshida H, Nagata M, Saito K, Wang KL, Ecker JR. Arabidopsis ETO1 specifically interacts with and negatively regulates type 2 1-aminocyclopropane-1-carboxylate synthases. BMC Plant Biol 2005; 5:14; 10.1186/1471-2229-5-14; PMID: 16091151
- Prasad ME, Schofield A, Lyzenga W, Liu H, Stone SL. Arabidopsis RING E3 ligase XBAT32 regulates lateral root production through its role in ethylene biosynthesis. Plant Physiol 2010; 153:1587 - 96; 10.1104/pp.110.156976; PMID: 20511490
- Li G, Meng X, Wang R, Mao G, Han L, Liu Y, et al. Dual-level regulation of ACC synthase activity by MPK3/MPK6 cascade and its downstream WRKY transcription factor during ethylene induction in Arabidopsis. PLoS Genet 2012; 8:e1002767; 10.1371/journal.pgen.1002767; PMID: 22761583
- Liu Y, Zhang S. Phosphorylation of ACC synthase by MPK6, a stress-responsive MAPK, induces ethylene biosynthesis in Arabidopsis. Plant Cell 2004; 16:3386 - 99; 10.1105/tpc.104.026609; PMID: 15539472
- Joo S, Liu Y, Lueth A, Zhang S. MAPK phosphorylation-induced stabilization of ACS6 protein is mediated by the non-catalytic C-terminal domain, which also contains the cis-determinant for rapid degradation by the 26S proteasome pathway. Plant J 2008; 54:129 - 40; 10.1111/j.1365-313X.2008.03404.x; PMID: 18182027
- Kamiyoshihara Y, Iwata M, Fukaya T, Tatsuki M, Mori H. Turnover of LeACS2, a wound-inducible 1-aminocyclopropane-1-carboxylic acid synthase in tomato, is regulated by phosphorylation/dephosphorylation. Plant J 2010; 64:140 - 50; PMID: 20659278
- Han L, Li GJ, Yang KY, Mao G, Wang R, Liu Y, et al. Mitogen-activated protein kinase 3 and 6 regulate Botrytis cinerea-induced ethylene production in Arabidopsis. Plant J 2010; 64:114 - 27; PMID: 20659280
- Choudhury SR, Roy S, Sengupta DNA. A Ser/Thr protein kinase phosphorylates MA-ACS1 (Musa acuminata 1-aminocyclopropane-1-carboxylic acid synthase 1) during banana fruit ripening. Planta 2012; 236:491 - 511; 10.1007/s00425-012-1627-9; PMID: 22419220
- Wang H, Mei W, Qin Y, Zhu Y. 1-Aminocyclopropane-1-carboxylic acid synthase 2 is phosphorylated by calcium-dependent protein kinase 1 during cotton fiber elongation. Acta Biochim Biophys Sin (Shanghai) 2011; 43:654 - 61; 10.1093/abbs/gmr056; PMID: 21742672
- Skottke KR, Yoon GM, Kieber JJ, DeLong A. Protein phosphatase 2A controls ethylene biosynthesis by differentially regulating the turnover of ACC synthase isoforms. PLoS Genet 2011; 7:e1001370; 10.1371/journal.pgen.1001370; PMID: 21533019
- Chang IF, Curran A, Woolsey R, Quilici D, Cushman JC, Mittler R, et al. Proteomic profiling of tandem affinity purified 14-3-3 protein complexes in Arabidopsis thaliana. Proteomics 2009; 9:2967 - 85; 10.1002/pmic.200800445; PMID: 19452453
- Ichimura K, Shinozaki K, Tena G, Sheen J, Henry Y, et al, MAPK Group. Mitogen-activated protein kinase cascades in plants: a new nomenclature. Trends Plant Sci 2002; 7:301 - 8; 10.1016/S1360-1385(02)02302-6; PMID: 12119167
- Kim CY, Liu Y, Thorne ET, Yang H, Fukushige H, Gassmann W, et al. Activation of a stress-responsive mitogen-activated protein kinase cascade induces the biosynthesis of ethylene in plants. Plant Cell 2003; 15:2707 - 18; 10.1105/tpc.011411; PMID: 14555690
- Colcombet J, Hirt H. Arabidopsis MAPKs: a complex signalling network involved in multiple biological processes. Biochem J 2008; 413:217 - 26; 10.1042/BJ20080625; PMID: 18570633
- Larsen PB, Cancel JD. Enhanced ethylene responsiveness in the Arabidopsis eer1 mutant results from a loss-of-function mutation in the protein phosphatase 2A A regulatory subunit, RCN1. Plant J 2003; 34:709 - 18; 10.1046/j.1365-313X.2003.01762.x; PMID: 12787251