Abstract
The minor chloroplastic O-acetylserine(thiol)lyase isoform encoded by the CS26 gene in Arabidopsis thaliana has been described as an S-sulfocysteine synthase enzyme that plays an important role in chloroplast function. This enzyme is located in the thylakoid lumen, and its S-sulfocysteine activity is essential for the proper photosynthetic performance of the chloroplast under long-day growth conditions. Based on the present knowledge of this enzyme, we suggest that S-sulfocysteine synthase functions as a protein sensor to detect the accumulation of thiosulfate as a result of the inadequate detoxification of reactive oxygen species generated under conditions of excess light to produce the S-sulfocysteine molecule that triggers protection mechanisms of the photosynthetic apparatus.
Cysteine is the first organic compound with reduced sulfur synthesized by plants in photosynthetic primary sulfate assimilation and occupies a central position in plant primary and secondary metabolism due to its biochemical functions. In plants, cysteine biosynthesis is accomplished by the sequential reaction of two enzymes: serine acetyltransferase (SAT), which catalyzes the synthesis of the intermediate product O-acetylserine from acetyl-CoA and serine, and O-acetylserine(thiol)lyase (OASTL), which incorporates the sulfide that is derived from the assimilatory reduction of sulfate to O-acetylserine, thus producing cysteine. These biosynthetic enzymes are encoded by members of a large gene family and are represented by five different SAT isoforms and eight OASTL isoforms in Arabidopsis thaliana.Citation1,Citation2
The OASTL isoforms belong to the superfamily of β-substituting alanine synthase enzymes; in Arabidopsis, the proteins encoded by the OAS-A1, OAS-B and OAS-C genes are authentic OASTLs and are the most abundant that drive cysteine biosynthesis in the cytosol, plastids and mitochondria, respectively.Citation3-Citation5 The OASTL family also includes the isoform encoded by ATCYS-C1, which functions as a β-cyanoalanine synthase enzyme that is involved in cyanide detoxification processes in mitochondria,Citation6,Citation7 and the cytosolic isoform encoded by DES1, which catalyzes the desulfuration of L-cysteine.Citation8
In bacteria, the biosynthesis of cysteine is accomplished by the isoenzymes A (encoded by cysK) and B (encoded by cysM); however, the latter is also able to incorporate thiosulfate to produce S-sulfocysteine. S-sulfocysteine biosynthesis has also been reported in several photosynthetic bacterial strains,Citation9 although this activity had not been previously reported in plants or eukaryotic microorganisms. Recently, the minor chloroplastic OASTL isoform encoded by the CS26 gene from Arabidopsis was described as an S-sulfocysteine synthase enzyme that plays an important role in chloroplast function.Citation10 Further characterization of knock-down mutants revealed that the loss of CS26 function resulted in dramatic phenotypic changes that were dependent on the photoperiod conditions. The photosynthetic characterization of the cs26 mutant under long-day growth conditions (LD) revealed significant reductions in most of the photosynthetic parameters, including the net CO2 assimilation rate, mesophyll conductance and mitochondrial respiration in darkness, and showed that cs26 under LD required more absorbed quanta per driven electron flux and fixed CO2. However, under short-day growth conditions (SD), the cs26 mutant behaves photosynthetically similarly to wild-type.Citation11
From the point of view of the anatomical and photosynthetic characteristics of leaves, the acclimation of Arabidopsis to SD and LD photoperiods mimics the responses observed in leaves acclimated to low light and medium/high light, respectively. The adaptation to high light requires that plants sense the light conditions and modify their transcript profile and metabolism to balance the production and detoxification of ROS.Citation12 Our work clearly shows that the absence of S-sulfocysteine synthase activity in chloroplasts disturbs the production and detoxification of ROS and prevents the plant from defending itself from the photochemical damage produced by the photosystems and the electron transport chain during LD photoperiod. Therefore, under low light, the cs26 mutant behaves as if it was growing under high-light conditions.Citation11
Although we cannot define the function of this enzyme in the chloroplast, we can describe how this enzyme differs from the other OASTL proteins with chloroplast localization. First, the CS26 protein has a lumenal location, in contrast to the OAS-B isoform, which is located in the stroma. Second, the protein has S-sulfocysteine synthase activity, incorporating thiosulfate instead of sulfide, as does the stromal isoform. These two features of CS26 raise two important issues: the conditions under which thiosulfate is generated in the chloroplast and the role that the enzyme and the generated product, S-sulfocysteine, plays in the thylakoid lumen. Our hypothesis about the role of CS26 and S-sulfocysteine in the chloroplasts is summarized in .
Figure 1. Schematic representation of the electron transfer chain from the Photosystem II (PSII) complex through the plastoquinol pool (PQH2), the proton pump cytochrome b6f, the Photosystem I (PSI) complex and, finally, to the electron carrier ferredoxin (Fd). Chloroplast sulfate assimilation depends on ferredoxin as a reductant of sulfite reductase, reducing sulfite to sulfide, which is then incorporated into the backbone of O-acetylserine (OAS) to form cysteine (CYS) in the chloroplast stroma. Under conditions of excess light, the light absorbed by the chloroplasts exceeds their photosynthetic capacity and can lead to the production of reactive oxygen species, such as singlet oxygen (1O2*), superoxide anion (O2-) and hydrogen peroxide, which may interfere with the reduction of sulfite or may oxidize sulfide to form thiosulfate. Thiosulfate acts as a CS26 substrate to generate S-sulfocysteine, which may act as a mild oxidant in the lumen, inducing redox changes to activate such regulatory proteins as violaxanthin de-epoxidase (VDE), which is required to activate the pH-dependent regulation of photosynthetic light harvesting (qE) to dissipate the excess of absorbed light energy as heat.
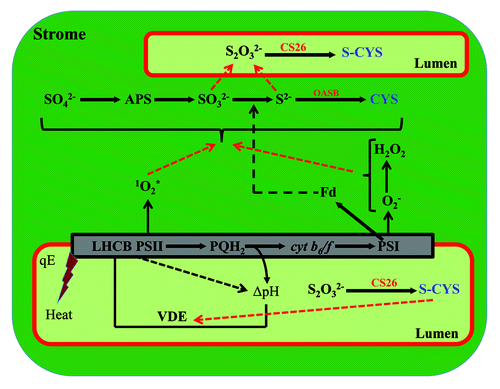
Light absorption by the Photosystem II (PSII) complex splits water into electrons, protons and oxygen and initiates the electron transport chain through the plastoquinol pool (PQH2), which carries the electrons to the proton pump cytochrome b6f, the Photosystem I (PSI) complex, and, ultimately, to the soluble electron carrier ferredoxin (Fd). Chloroplast sulfate assimilation depends on ferredoxin as the reductant of sulfite reductase, reducing sulfite to sulfide, which is then incorporated into the backbone of O-acetylserine to form cysteine. Under conditions of excess light, the light absorbed by the chloroplasts exceeds their photosynthetic capacity and can lead to the production of reactive oxygen species, such as singlet oxygen (1O2*), superoxide anion (O2-) and hydrogen peroxide, which may interfere with the reduction of sulfite or may oxidize sulfide to form thiosulfate. Thiosulfate serves as a CS26 substrate to generate S-sulfocysteine in the lumen, acting as a warning sign of possible oxidative stress. As it would be necessary to activate defense mechanisms under these circumstances, we postulate that S-sulfocysteine may act as a mild oxidant in the lumen, inducing redox changes to activate regulatory proteins by upregulating the oxidation of the Cys residues of the lumenal proteins with activities modified by these oxidation eventsCitation13 and protecting the photosynthetic apparatus from photo-oxidative damage. Some potential proteins targets of S-Cys-dependent oxidation are violaxanthin de-epoxidase (VDE), an enzyme that converts violaxanthin to antheraxanthin and zeaxanthin as part of the xanthophyll cycle and is required to activate the pH-dependent regulation of photosynthetic light harvesting to dissipate excess absorbed light energy as heatCitation14 (). VDE has been identified as a thioredoxin target, and its reduction inactivates the enzymatic activity of the protein in vitro.Citation15
In conclusion, we suggest that S-sulfocysteine synthase may function as a protein sensor to detect the accumulation of thiosulfate that is the result of the inadequate detoxification of ROS, forming the S-sulfocysteine molecule, which triggers protection mechanisms of the photosynthetic apparatus.
Acknowledgments
We would like to thank the European Regional Development Fund and the Ministerio de Economía y Competitividad (grant no. BIO2010-15201 and CSD2007-00057) Spain, and Junta de Andalucia (CVI-7190) for their financial support.
Disclosure of Potential Conflicts of Interest
No potential conflicts of interest were disclosed.
References
- Howarth JR, Domínguez-Solís JR, Gutiérrez-Alcalá G, Wray JL, Romero LC, Gotor C. The serine acetyltransferase gene family in Arabidopsis thaliana and the regulation of its expression by cadmium. Plant Mol Biol 2003; 51:589 - 98; http://dx.doi.org/10.1023/A:1022349623951; PMID: 12650624
- Takahashi H, Kopriva S, Giordano M, Saito K, Hell R. Sulfur assimilation in photosynthetic organisms: molecular functions and regulations of transporters and assimilatory enzymes. Annu Rev Plant Biol 2011; 62:157 - 84; http://dx.doi.org/10.1146/annurev-arplant-042110-103921; PMID: 21370978
- López-Martín MC, Becana M, Romero LC, Gotor C. Knocking out cytosolic cysteine synthesis compromises the antioxidant capacity of the cytosol to maintain discrete concentrations of hydrogen peroxide in Arabidopsis. Plant Physiol 2008; 147:562 - 72; http://dx.doi.org/10.1104/pp.108.117408; PMID: 18441224
- Heeg C, Kruse C, Jost R, Gutensohn M, Ruppert T, Wirtz M, et al. Analysis of the Arabidopsis O-acetylserine(thiol)lyase gene family demonstrates compartment-specific differences in the regulation of cysteine synthesis. Plant Cell 2008; 20:168 - 85; http://dx.doi.org/10.1105/tpc.107.056747; PMID: 18223034
- Watanabe M, Kusano M, Oikawa A, Fukushima A, Noji M, Saito K. Physiological roles of the beta-substituted alanine synthase gene family in Arabidopsis. Plant Physiol 2008; 146:310 - 20; http://dx.doi.org/10.1104/pp.107.106831; PMID: 18024555
- García I, Castellano JM, Vioque B, Solano R, Gotor C, Romero LC. Mitochondrial beta-cyanoalanine synthase is essential for root hair formation in Arabidopsis thaliana. Plant Cell 2010; 22:3268 - 79; http://dx.doi.org/10.1105/tpc.110.076828; PMID: 20935247
- Álvarez C, García I, Romero LC, Gotor C. Mitochondrial Sulfide Detoxification Requires a Functional Isoform O-Acetylserine(thiol)lyase C in Arabidopsis thaliana. Mol Plant 2012; 5:1217 - 26; http://dx.doi.org/10.1093/mp/sss043; PMID: 22511607
- Alvarez C, Calo L, Romero LC, García I, Gotor C. An O-acetylserine(thiol)lyase homolog with L-cysteine desulfhydrase activity regulates cysteine homeostasis in Arabidopsis. Plant Physiol 2010; 152:656 - 69; http://dx.doi.org/10.1104/pp.109.147975; PMID: 19955263
- Hensel G, Trüper HG. Cysteine and S-sulfocysteine biosynthesis in phototrophic bacteria. Arch Microbiol 1976; 109:101 - 3; http://dx.doi.org/10.1007/BF00425119; PMID: 962465
- Bermúdez MA, Páez-Ochoa MA, Gotor C, Romero LC. Arabidopsis S-sulfocysteine synthase activity is essential for chloroplast function and long-day light-dependent redox control. Plant Cell 2010; 22:403 - 16; http://dx.doi.org/10.1105/tpc.109.071985; PMID: 20179139
- Bermúdez MA, Galmés J, Moreno I, Mullineaux PM, Gotor C, Romero LC. Photosynthetic adaptation to length of day is dependent on S-sulfocysteine synthase activity in the thylakoid lumen. Plant Physiol 2012; 160:274 - 88; http://dx.doi.org/10.1104/pp.112.201491; PMID: 22829322
- Lepistö A, Rintamäki E. Coordination of plastid and light signaling pathways upon development of Arabidopsis leaves under various photoperiods. Mol Plant 2012; 5:799 - 816; http://dx.doi.org/10.1093/mp/ssr106; PMID: 22199239
- Buchanan BB, Luan S. Redox regulation in the chloroplast thylakoid lumen: a new frontier in photosynthesis research. J Exp Bot 2005; 56:1439 - 47; http://dx.doi.org/10.1093/jxb/eri158; PMID: 15851415
- Li Z, Wakao S, Fischer BB, Niyogi KK. Sensing and responding to excess light. Annu Rev Plant Biol 2009; 60:239 - 60; http://dx.doi.org/10.1146/annurev.arplant.58.032806.103844; PMID: 19575582
- Hall M, Mata-Cabana A, Åkerlund H-E, Florencio FJ, Schröder WP, Lindahl M, et al. Thioredoxin targets of the plant chloroplast lumen and their implications for plastid function. Proteomics 2010; 10:987 - 1001; PMID: 20049866