Abstract
With the exception of the grasses, plants rely on a reduction-based iron (Fe) uptake system that is compromised by high soil pH, leading to severe chlorosis and reduced yield in crop plants. We recently reported that iron deficiency triggers the production of secondary metabolites that are beneficial for Fe uptake in particular at high external pH when iron is present but not readily available. The exact function of these metabolites, however, remains enigmatic. Here, we speculate on the mechanism by which secondary metabolites secreted by roots from Fe-deficient plants improve Fe acquisition. We suggest that the production and excretion of Iron Binding Compounds (IBCs) constitute an integrative, pH-insensitive component of the reduction-based iron uptake strategy in plants.
Keywords: :
Although iron (Fe) is generally present in large amounts, in aerobic soils Fe tends to form oxyhydrates of low solubility, in particular at neutral or high pH. When atmospheric oxygen increased as a result of the evolution of photosynthesis, plants were forced to evolve strategies that improve Fe acquisition, which was chiefly achieved by increasing the solubility of immobile Fe pools. All plant species except the grasses have employed a mechanism that includes reduction of ferric chelates, mediated by a plasma membrane-bound oxidoreductase (FRO2 in Arabidopsis).Citation1 The reduction of Fe(III) weakens the stability of the chelate and the released Fe(II) is taken up by a ZIP family transporter (IRT1).Citation2 A drawback of this strategy is that it works best at a slightly acidic pH and is strongly impaired in soils with high pH.Citation3 This is partly counteracted by induction of a P-type H+-ATPase that provides a favorable pH environment (AHA2).Citation4 Excretion of organic compounds, mainly phenolics or flavins, have been associated with the Fe deficiency response since the early seventies,Citation5,Citation6 but were later considered to be of minor importance and, with some notable exceptions (e.g., the massive production of riboflavin and riboflavin derivatives by some species),Citation7,Citation8 were largely neglected in studies related to iron acquisition processes in model species such as Arabidopsis. Nevertheless, the frequent observation of this phenomenon led to speculations on the function of these compounds in Fe acquisition, comprising effects on the microbiome in the rhizosphere, a function (for flavins) in electron transport during the reduction of Fe(III), chelation of Fe, and chemical reduction of Fe(III). The first clear-cut evidence for a function of Fe deficiency-induced root secretions in Fe uptake was provided by Jin et al. showing that phenolics secreted by red clover roots facilitated the acquisition of Fe bound to anionic residues in the apoplast.Citation9 Similarly, transgenic rice plants overexpressing the phenolic efflux transporter PEZ1 grew better on soils with high pH and poor Fe solubility.Citation10 In Arabidopsis, robust induction of the phenylpropanoid pathway, both at the transcript and protein levels,Citation11,Citation12 indicating that in roots of Fe-deficient plants phenolic compounds are produced in large amounts. Mutations in a key step in the pathway, the formation of 6’hydroxy-feruloyl-CoA from feruloyl-CoA by the oxygenase AtF6’H1 (At3g13610), and disruption of a putative phenolic efflux transporter, PDR9, compromised the excretion of fluorescent phenolics and growth on media with insoluble Fe.Citation13 An independent study extended our findings by identifying the secreted phenolics in Arabidopsis as non-glycosylated members of the coumarin family.Citation14 Notably, three steps in the phenylproanoid pathway and the expression of PDR9 are controlled by the master regulator FIT, a bHLH-type transcription factor that regulates the expression level of IRT1, FRO2 and AHA2.Citation15,Citation16 A comparison of the changes in the Arabidopsis transcriptome with those in Medicago truncatula suggested that the induction of the pathways leading to production of phenolics (in Arabidopsis roots) and flavins (in Medicago roots) is mutually exclusive.Citation16 Notably, flavins secreted by Medicago roots rescued the phenotype of pdr9–2 and f6’h1–1 mutants as efficiently as Arabidopsis-derived phenolics, indicating that the two compounds play similar roles in Fe acquisition. While other functions cannot be excluded, together these data suggest that the main role of secreted IBCs lies in the mobilization of otherwise insoluble Fe pools. Possible scenarios of how IBCs improve Fe uptake are shown in . A plausible function of IBCs is the formation of Fe(III)-IBC chelates from Fe(III) oxyhydrates that can then serve as a substrate for FRO2-mediated ferric reduction (). Iron chelating properties have been shown for both coumarins and flavins.Citation17,Citation18 It is interesting to note that at both feruloyl-CoA and riboflavin a hydroxyl group is added in the last step of the biosynthetic pathway that likely improves solubility of the secreted compound. Alternatively, the main function of IBCs could be related to the reduction of ferric iron, acting as long distance electron shuttle (). Theoretically, the midpoint potential of flavins and coumarins allows direct reduction of most Fe(III) oxides. Such a mechanism has been described for flavins secreted by bacteria.Citation19-Citation21 A scenario that would explain old and new findings on ‘reductants’ released by plant roots implies that ICBs are only important for the reduction of insoluble Fe compounds, but are not crucial when Fe(III) is soluble, as with many Fe(III) chelates. For bacteria, such a scenario is supported by a recent study, which demonstrates that in Shewanella oneidensis strains defective in the bacterial flavin adenine dinucleotide exporter bfe, the amount of extracellular flavins and the rate of reduction of insoluble Fe(III) oxides were reduced, while the reduction of soluble Fe(III) was unaffected.Citation22 To work efficiently, oxidized IBCs need to be recycled by reduction, likely by FRO2 or by other electron transport systems in the plasma membrane. Chelation and reduction are not mutually exclusive functions of IBCs and might in combination provide support for a strongly compromised FRO2/IRT1-based Fe acquisition at high pH. It can also not be excluded that, similar to the Fe uptake system of the grasses, an Fe-IBC chelate is taken up as such, circumventing the FRO2/IRT1 system (). Such a scenario appears plausible against the recent findings that the two Fe uptake strategies are not mutually exclusive.Citation23,Citation24 For example, rice, a graminaceous species that does not rely on reduction and takes up Fe(III) bound to plant-borne phytosiderophores, possess a functional IRT1 ortholog that transports Fe(II) into the cell when present in sufficient amounts, for example in waterlogged soils.Citation24 However, since knockout of FRO2 and IRT1 result in severe phenotypes, we suggest that such a mechanism could only represent a supportive mechanism that comes into play when Fe is not readily available, for example at high pH.
Figure 1. Schematic depiction of possible functions of iron binding compounds (IBCs). (A) Chelation of Fe(III) mobilized from Fe(III) oxide with subsequent reduction of Fe(III) by FRO2. (B). Reduction of Fe(III) by reduced IBCs and subsequent recycling of the redox state via reduction of IBCs by FRO2 or other oxidoreductases. (C) IBCs that function in an independent Fe uptake system. After secretion, IBCs bind Fe(III) released from Fe(III) oxides and the Fe(III)-IBC complex is taken up as such without prior reduction. IBCOx, oxidized IBC; IBCRed, reduced IBC. Symbols represent proteins identified in Arabidopsis but functional orthologs may exist in other species.
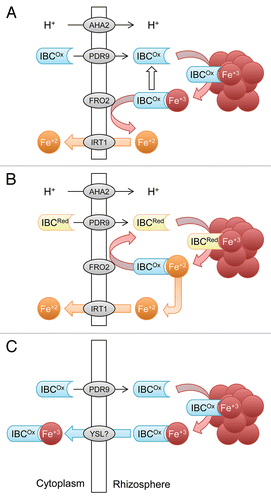
While the exact physiological function of flavins and phenolics in Fe uptake and the molecular mechanisms underlying these functions remains to be experimentally dissected, it can be concluded that the excretion of IBCs constitutes an integral component of the reduction-based Fe acquisition strategy that becomes crucial when the activity of free Fe is very low, i.e., at high pH. This assumption is supported by the tight co-expression of the genes mediating the production of IBCs with genes with critical functions in Fe uptake and the regulation of the pathways by AtFIT/MtFIT.Citation13 Secretion of IBCs is an effective mechanism to improve Fe uptake, and manipulation of the production/excretion of IBCs may represent a promising way to combat Fe deficiency-induced anemia.
Disclosure of Potential Conflicts of Interest
No potential conflicts of interest were disclosed.
Acknowledgments
We thank Thomas J Buckhout (Humboldt University) for valuable suggestions and critical comments on the manuscript. Work in the Schmidt laboratory is supported by grants from Academia Sinica and NSC.
References
- Robinson NJ, Procter CM, Connolly EL, Guerinot ML. A ferric-chelate reductase for iron uptake from soils. Nature 1999; 397:694 - 7; http://dx.doi.org/10.1038/17800; PMID: 10067892
- Eide D, Broderius M, Fett J, Guerinot ML. A novel iron-regulated metal transporter from plants identified by functional expression in yeast. Proc Natl Acad Sci U S A 1996; 93:5624 - 8; http://dx.doi.org/10.1073/pnas.93.11.5624; PMID: 8643627
- Schmidt W. Mechanisms and regulation of reduction-based iron uptake in plants. New Phytol 1999; 141:1 - 26; http://dx.doi.org/10.1046/j.1469-8137.1999.00331.x
- Santi S, Schmidt W. Dissecting iron deficiency-induced proton extrusion in Arabidopsis roots. New Phytol 2009; 183:1072 - 84; http://dx.doi.org/10.1111/j.1469-8137.2009.02908.x; PMID: 19549134
- Brown JC, Ambler JE. Reductants released by roots of Fe-deficient soybeans. Agron J 1973; 65:311 - 4; http://dx.doi.org/10.2134/agronj1973.00021962006500020037x
- Römheld V, Marschner H. Mechanism of iron uptake by peanut plants : I. Fe reduction, chelate splitting, and release of phenolics. Plant Physiol 1983; 71:949 - 54; http://dx.doi.org/10.1104/pp.71.4.949; PMID: 16662934
- Susín S, Abián J, Sánchez-Baeza F, Peleato ML, Abadía A, Gelpí E, Abadía J. Riboflavin 3′- and 5′-sulfate, two novel flavins accumulating in the roots of iron-deficient sugar beet (Beta vulgaris). J Biol Chem 1993; 268:20958 - 65; PMID: 8407931
- Rodríguez-Celma J, Vázquez-Reina S, Orduna J, Abadía A, Abadía J, Álvarez-Fernández A, López-Millán AF. Characterization of flavins in roots of Fe-deficient strategy I plants, with a focus on Medicago truncatula.. Plant Cell Physiol 2011; 52:2173 - 89; http://dx.doi.org/10.1093/pcp/pcr149; PMID: 22039102
- Jin CW, You GY, He YF, Tang C, Wu P, Zheng SJ. Iron deficiency-induced secretion of phenolics facilitates the reutilization of root apoplastic iron in red clover. Plant Physiol 2007; 144:278 - 85; http://dx.doi.org/10.1104/pp.107.095794; PMID: 17369430
- Ishimaru Y, Kakei Y, Shimo H, Bashir K, Sato Y, Sato Y, Uozumi N, Nakanishi H, Nishizawa NK. A rice phenolic efflux transporter is essential for solubilizing precipitated apoplasmic iron in the plant stele. J Biol Chem 2011; 286:24649 - 55; http://dx.doi.org/10.1074/jbc.M111.221168; PMID: 21602276
- Yang TJ, Lin WD, Schmidt W. Transcriptional profiling of the Arabidopsis iron deficiency response reveals conserved transition metal homeostasis networks. Plant Physiol 2010; 152:2130 - 41; http://dx.doi.org/10.1104/pp.109.152728; PMID: 20181752
- Lan P, Li W, Wen TN, Shiau JY, Wu YC, Lin W, Schmidt W. iTRAQ protein profile analysis of Arabidopsis roots reveals new aspects critical for iron homeostasis. Plant Physiol 2011; 155:821 - 34; http://dx.doi.org/10.1104/pp.110.169508; PMID: 21173025
- Rodríguez-Celma J, Lin WD, Fu GM, Abadía J, López-Millán AF, Schmidt W. Mutually exclusive alterations in secondary metabolism are critical for the uptake of insoluble iron compounds by Arabidopsis and Medicago truncatula.. Plant Physiol 2013; 162:1473 - 85; http://dx.doi.org/10.1104/pp.113.220426; PMID: 23735511
- Fourcroy P, Sisó-Terraza P, Sudre D, Savirón M, Reyt G, Gaymard F, et al. Involvement of the ABCG37 transporter in secretion of scopoletin and derivatives by Arabidopsis roots in response to iron deficiency. New Phytol 2013;
- Colangelo EP, Guerinot ML. The essential basic helix-loop-helix protein FIT1 is required for the iron deficiency response. Plant Cell 2004; 16:3400 - 12; http://dx.doi.org/10.1105/tpc.104.024315; PMID: 15539473
- Ivanov R, Brumbarova T, Bauer P. Fitting into the harsh reality: regulation of iron-deficiency responses in dicotyledonous plants. Mol Plant 2012; 5:27 - 42; http://dx.doi.org/10.1093/mp/ssr065; PMID: 21873619
- Albert A. Quantitative studies of the avidity of naturally occurring substances for trace metals. III. Pteridines, riboflavin and purines. Biochem J 1953; 54:646 - 54; PMID: 13058968
- Mladenka P, Macáková K, Zatloukalová L, Reháková Z, Singh BK, Prasad AK, Parmar VS, Jahodár L, Hrdina R, Saso L. In vitro interactions of coumarins with iron. Biochimie 2010; 92:1108 - 14; http://dx.doi.org/10.1016/j.biochi.2010.03.025; PMID: 20381579
- von Canstein H, Ogawa J, Shimizu S, Lloyd JR. Secretion of flavins by Shewanella species and their role in extracellular electron transfer. Appl Environ Microbiol 2008; 74:615 - 23; http://dx.doi.org/10.1128/AEM.01387-07; PMID: 18065612
- Marsili E, Baron DB, Shikhare ID, Coursolle D, Gralnick JA, Bond DR. Shewanella secretes flavins that mediate extracellular electron transfer. Proc Natl Acad Sci U S A 2008; 105:3968 - 73; http://dx.doi.org/10.1073/pnas.0710525105; PMID: 18316736
- Brutinel ED, Gralnick JA. Shuttling happens: soluble flavin mediators of extracellular electron transfer in Shewanella. Appl Microbiol Biotechnol 2012; 93:41 - 8; http://dx.doi.org/10.1007/s00253-011-3653-0; PMID: 22072194
- Kotloski NJ, Gralnick JA. Flavin electron shuttles dominate extracellular electron transfer by Shewanella oneidensis. MBio 2013; 4:e00553 - 12; http://dx.doi.org/10.1128/mBio.00553-12; PMID: 23322638
- Buckhout T, Schmidt W.. Iron in plants. eLS 2013.
- Kobayashi T, Nishizawa NK. Iron uptake, translocation, and regulation in higher plants. Annu Rev Plant Biol 2012; 63:131 - 52; http://dx.doi.org/10.1146/annurev-arplant-042811-105522; PMID: 22404471