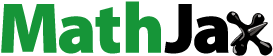
Abstract
The Venus flytrap (Dionaea muscipula Ellis) captures insects with one of the most rapid movements in the plant kingdom. We investigated trap closure by mechanical and electrical stimuli using the novel charge-injection method and high-speed recording. We proposed a new hydroelastic curvature mechanism, which is based on the assumption that the lobes possess curvature elasticity and are composed of outer and inner hydraulic layers with different hydrostatic pressure. The open state of the trap contains high elastic energy accumulated due to the hydrostatic pressure difference between the hydraulic layers of the lobe. Stimuli open pores connecting the two layers, water rushes from one hydraulic layer to another, and the trap relaxes to the equilibrium configuration corresponding to the closed state. In this paper we derived equations describing this system based on elasticity Hamiltonian and found closing kinetics. The novel charge-injection stimulation method gives insight into mechanisms of the different steps of signal transduction and response in the plant kingdom.
Introduction
Most plants respond to mechanical stimuli and those with rapid and highly noticeable touch-stimulus response have received much attention, such as the Venus flytrap (Dionaea muscipula Ellis). Touching trigger hairs, protruding from the upper leaf epidermis of the Venus flytrap, activates mechanosensitive ion channels and generates receptor potentials,Citation1,Citation2 which can induce action potentials.Citation3–Citation8 It was found that two action potentials are required to trigger the trap closing.Citation2,Citation4,Citation9
The history of studying the Venus flytrap spans more than a century. Although the sequence of actions is clearly described in the existing literature, the exact mechanism of the trap closure is still poorly understood. Indeed, quite a bit is known about how the flytrap closes: stimulating the trigger hair twice within 40 s unleashes two action potentials triggering curvature changes, which helps the plant rapidly close its upper leaf. When trigger hairs in the open trap receive mechanical stimuli, a receptor potential is generated.Citation10,Citation11 Two mechanical stimuli are required for closing the trap in vivo.Citation12,Citation13 However, at high temperatures (36–40°C) only one stimulus is required for trap closure.Citation13 Receptor potentials generate action potentials,Citation1–Citation4,Citation14 which can propagate in the plasmodesmata of the plant to the midrib.Citation4 Uncouplers and blockers of fast anion and potassium channels can inhibit action potential propagation in the Venus flytrap.Citation2,Citation4,Citation6,Citation15 The trap accumulates the electrical charge delivered by an action potential. Once a threshold value of the charge is accumulated, ATP hydrolysisCitation16 and fast proton transport start,Citation17,Citation18 and aquaporin opening is initiated.Citation2 Fast proton transport induces transport of water and a change in turgor.Citation7
A number of contradictory models were proposed,Citation7,Citation9,Citation12,Citation14,Citation18–Citation22 and still there is no agreement between the researchers.Citation7 Recently, the focus of interest returned to the original ideas proposed by DarwinCitation12 in the 19th century. In his seminal work, DarwinCitation12 demonstrated that the basic catching movement of the Venus flytrap involves the transformation of the leaf curvature from convex to concave resulting in the closing of the trap. DarwinCitation12 wrote: “We know that the lobes, whilst closing, become slightly incurved throughout their whole breadth. This movement appears to be due to the contraction of the superficial layers of cells over the whole upper surface. In order to observe their contraction, a narrow strip was cut out of one lobe at right angles to the midrib, so that the surface of the opposite lobe could be seen in this part when the leaf was shut. After the leaf had recovered from the operation and had re-expanded, three minute black dots were made on the surface opposite to the slit or window, in a line at right angles to the midrib. The distance between the dots was found to be 40/1000 of an inch, so that the two extreme dots were 80/1000 of an inch apart. One of the filaments was now touched and the leaf closed. On again measuring the distances between the dots, the two next to the midrib were nearer together by 1 to 2/1000 of an inch, and the two further dots by 3 to 4/1000 of an inch, than they were before; so that the two extreme dots now stood about 5/1000 of an inch (0.127 mm) nearer together than before. If we suppose the whole upper surface of the lobe, which was 400/1000 of an inch in breadth, to have contracted in the same proportion, the total contraction will have amounted to about 25/1000 or 1/40 of an inch (0.635 mm).”
DarwinCitation12 established that the upper leaf of the Venus flytrap includes two distinct layers of cells at upper and lower surfaces that behave quite differently in the process of trap closure. The finding of these two independent layers was later confirmed by many authors and their role was related to the turgor pressure.Citation23–Citation25 It is well known that some functions in plants and fungi can only be driven by exploiting hydrodynamic flow, such as stomata guard cell opening and closing, leaf pulvini motor organ, mechanical traps of carnivorous plants, and fungal appressorial penetration.Citation26–Citation28
It is common knowledge that the leaves of the Venus flytrap actively employ turgor pressure and hydrodynamic flow for fast movement and catching insects. In these processes the upper and lower surfaces of the leaf behave quite differently. The loss of turgor by parenchyma, lying beneath the upper epidermis, accompanied by the active expansion of the tissues of the lower layers of parenchyma near the lower epidermis closes the trap.Citation9,Citation12,Citation13,Citation29,Citation30 The cells on the inner face of the trap jettison their cargo of water, shrink and allow the trap lobe to fold over. The cells of the lower epidermis expand rapidly, folding the trap lobes over.Citation9
Recently, Forterre et al.,Citation19 reproduced the DarwinCitation12 work at the modern technical level with high-speed video imaging and non-invasive microscopy techniques. Forterre et al.,Citation19 documented in minute details the change of the geometry of the leaf in two dimensions and brought up the idea that elastic energy might play an important role in the closing of the trap.
The mechanical and anatomical features mentioned above constitute the basis of the proposed hydroelastic curvature model. It includes two layers of cells at the leaf of the Venus flytrap with different turgor pressure,Citation23–Citation25 the rush of water from one layer to another,Citation9,Citation12,Citation13,Citation29,Citation30 the existence of natural curvature in the leafCitation12 that changes with water exchange and consecutive relaxation of the leaf to the closed configuration.
Earlier, we found that the trap of the Venus flytrap can be also closed by electrical stimulation of a midrib.Citation2,Citation4 Here we present detailed experiments for comparative study of mechanical and electrical triggering of the trap and a new model of the closing mechanism.
Results
Theoretical: hydroelastic curvature model.
In the mechanical model of Forterre et al.,Citation19 the closing process of the Venus flytrap is described as a relaxation of the leaf to the new equilibrium state after triggering. They wrote: “Upon stimulation, the plant ‘actively’ changes one of its principal natural curvatures, the microscopic mechanism for which remains poorly understood.” We are going to address this issue and to elucidate what causes the change of spontaneous curvature of the leaf.
Our data suggest that elastic energy does play an important role, but closing the trap also involves another process that determines the transformation from an open to a closed state. For this purpose we propose the hydroelastic model that includes bending elasticity, turgor pressure and water jets. The closure of the Venus flytrap represents the nonmuscular movement based on hydraulics and mechanical elasticity. The nastic movements in various plants involve a large internal pressure (turgor) actively regulated by plants.
The leaf of the Venus flytrap is visualized as a thin, weakly-curved elastic shell with principal natural curvatures that depend on the hydrostatic state of the two surface layers of cell A and B (), where different hydrostatic pressures PA and PB are maintained.
Two layers of cells, mechanically connected to each other, behave like a bilayer couple where the in-plane expansion or contraction of any of them causes the change of curvature of the whole leaf. The bilayer couple hypothesis was first introduced by Sheetz and Singer.Citation31 They noticed that the proteins and the phospholipids of membranes are asymmetrically distributed in the two halves of the bilayer, which is most substantial for the erythrocyte membrane.
The two halves of the closed membrane bilayer may respond differently to various perturbations while remaining coupled to one another. One half of the bilayer may expand in the plane of the membrane relative to the other half of the bilayer, while the two layers remain in contact with one another. This leads to various functional consequences, including shape changes of the intact cell. This concept is called the bilayer couple hypothesis because of the analogy to the response of a bimetallic couple to changes in temperature. It remains very popular and applied to explanation of numerous phenomena, such as red blood cell transformations (see for example Lim et al.,Citation32 and references within) and the gating of mechanosensitive channels.Citation33
The bilayer couple properties were also extensively studied in connection with bilayer fusion, fission, endo- and exocytosis.Citation34,Citation35 We applied this technique for the design and analysis of the hydroelastic curvature model of the Venus flytrap. The model is based on the assumption that the driving force of the closing is the elastic curvature energy stored and locked in the leaves due to pressure differential between the outer and inner layers of the leaf ().
Unequal expansion of individual layers A and B results in bending of the leaf, and it will be described in terms of bending elasticity. Unequal expansion means that the torque M appears in the leaf. The energy of the bent layer A is described by the equation:
1
1
Here CAM is the total curvature of the layer A, CAG is the Gaussian curvature, CA0 is the spontaneous or intrinsic curvature of the layer, and κ designates the elasticity. Usually spontaneous curvature of layers is considered a constant—bA, depending on the composition of the layer, and describes the intrinsic tendency of the layer to bend. There is an additional source of bending—different pressure in two adjacent layers. One can easily visualize a number of mechanical models in which spontaneous curvature is proportional to the pressure. We shall not go into these details just assuming that CA0 = aAPA + bA. The same equations are valid for layer B.
The geometrical mean and Gaussian curvatures are defined as CAM = 1/R1 + 1/R2 and CAG = 1/(R1R2), where R1 and R2 are the main radii of curvature of the layer. We shall approximate the leaf by a spherical surface; then CAM = 2/R and CAG = CAM2/4. The leaf is thin and hence the two layers have the mean curvatures that are equal in absolute value but have opposite signs: CAM = −CBM = CM Total elastic energy of the lobe can be presented as:
2
2
Here, the coefficients aA and aB are assumed to be equal to a.
At the given pressures PA and PB, the equilibrium value of the mean curvature can be found from the minimum value of elastic energy (Equation22
2 ):
3
3
Here bL designates the difference between two intrinsic curvatures, bL = bA − bB. This equilibrium shape is maintained if the pressure difference does not change.
In the open state the pressure in the upper layer is higher than in the lower layer, maintaining the convex shape of the leaf. The fact that the hydrostatic pressure in different parts of the plant can be different is very well known. It is also knownCitation36 that stimulation of a Mimosa plant causes very fast redistribution of water. The authorsCitation36 found that after stimulation, water in the lower half of the main pulvinus is transferred to the upper half of the main pulvinus. Movement of the water in conjunction with Mimosa movement was visualized by a non-invasive NMR imaging procedure.Citation37 This fast water redistribution is obviously driven by the pressure difference between different parts of the plant and exchange occurs through open pores. Unfortunately, the anatomy and the nature of these pores are not known at the present time. Therefore, for the mechanical analysis we simply accept their existence.
We assume that water pores between the two hydraulic layers are closed at the resting state. The external trigger, either mechanical or electrical, results in the opening of these connecting pores; water rushes from the upper to the lower layer, the bilayer couple quickly changes its curvature from convex to concave and the trap closes.
If the trigger reaches threshold value at the moment ts and the characteristic time of the opening kinetics is τa then the open probability of the pores (after t ≥ ts) will be given by nop(t) = 1 − Exp[−(t − ts)/τa]. The rate of fluid transfer can be presented as J = nopL(PA − PB), where L is the hydraulic coefficient of pore permeability. If the pressure in the layer is proportional to the amount of fluid confined in it, the pressure will change with a rate proportional to the fluid transfer between the layers: dPA/dt = −krJ = −dPB/dt. This means that the sum of the two pressures remains constant: PA + PB = const = Ptotal. Then the variation of pressure is described by the equation:
4
4
Here the characteristic time of fluid transfer, τr = 1/(2 krL), is introduced. A similar equation for the mean curvature can be easily obtained from eqs. (Equation3
3
3 ) and (Equation4
4
4 ):
5
5
Initial curvature C1 in the open state can be introduced arbitrarily, while the final curvature C2 in the closed state is found from eq. (Equation5
5
5 ) automatically:
When solving this equation, one has to have in mind that the open probability nop is the function of time found above. If initial mean curvature at the moment t = ts is CM = C1, the solution of eq. (Equation55
5 ) at t ≥ ts is
6
6
The change in the shape of the Venus flytrap leaves according to the hydroelastic curvature model is presented in . It is very close to the pictures we recorded in .
At these recordings we measured the change of the distance, X, between the edges of the leaves of the Venus flytrap. The equation for this distance can be derived from as follows. If the area of the leaf is S, the distance between the edges is given by:
7
7
If the initial distance is X1, and the final distance is X2, the normalized distance is defined as x = X/X1. One can see that both distance and mean curvature of the leaf are described by the same function of time.
The trigger signal opens the pores between these layers and the fluid rushes from one layer to another. The leaf relaxes to its equilibrium state corresponding to the closed configuration. The distance between the edges of the trap was found to vary with time as:
8
8
This function was used for curve fitting in and the variation of curvature in this process is illustrated in , with parameters τa = 20 ms, τr = 70 ms and C2 = −C1. At this plot the Gaussian curvature is presented as the ratio CG/CG1. One can see that the mean curvature changes from positive to negative values in the process of trap closure while the Gaussian curvature never becomes negative: it decreases in the beginning, reaches zero, and then increases. In the specific case presented at this plot the Gaussian curvature returns to the initial value. The process of closing in this model and variation of the leaf curvature are presented in , where the case of zero Gaussian curvature corresponds to the B.
Experimental testing of the model.
The Venus flytrap can be closed by mechanical stimulation of trigger hairs using a cotton thread or a small piece of gelatin ( and B). The Venus flytrap also closed when we applied an electrical pulse between the midrib and a lobe of the upper leaf without mechanical stimulation. A charge-injection method has been used to precisely estimate the amount of electrical charge necessary to cause the closing of the leaves. demonstrates the closing of the Venus flytrap in 0.3 s after electrical stimulation with a positive electrode connected to the midrib and a negative electrode located in one of lobes. The inverted polarity pulse was not able to close the plant, and the closed trap would not open by electrical stimulus lasting up to 100 s.
A single electrical pulse exceeding a threshold (mean 13.63 µC, median 14.00 µC, std. dev. 1.51 µC, n = 41) causes closure of a trap and induces an electrical signal propagating between the lobes and the midrib.Citation2,Citation4 When charges are smaller, the trap will not close. Repeated application of small charges demonstrates a summation of stimuli. Two or more injections of electrical charges within a period of less then 50 s closes the trap as soon as a total of 14 µC charge is applied. Trap closing by electrical stimulus obeys the all-or-none law: there is no reaction for stimulus under the threshold and the speed of closing does not depend on stimulus strength above threshold.
Experimental points in show the kinetics of closing the upper leaf induced by mechanical or electrical stimuli. Closing consists of three distinctive phases (). Immediately after stimulation, there is a mechanically silent period with no observable movement of the plant. This is followed by a period when the movement of the lobes starts with acceleration. The third period of fast movement is actual trapping when the leaves quickly relax to the new equilibrium state.
Discussion
The goal of the hydroelastic curvature model was to describe the three stages of the Venus flytrap closing we discovered in our experiment and to try to elucidate the processes that govern these stages. We deliberately decided to make it as simple as possible so that the mechanical details would not mask the basic ideas. So, we visualized the leaf as a part of a spherical surface, though its actual shape is a little more complicated.Citation12,Citation19
The new hydroelastic curvature model is based on the assumption that the trap possesses curvature elasticity and consists of outer and inner hydraulic layers where different hydrostatic pressure can build up. The open state of the trap contains high elastic energy accumulated due to the hydrostatic pressure difference between the outer and inner layers of the lobe. Stimuli open pores connecting the two layers, water rushes from one hydraulic layer to another and the trap relaxes to the equilibrium configuration corresponding to the closed state. Water transport leads to extension of the cells on the exterior surface of the leaf and to a change in the natural curvature of the leaf, which is likely to drive the closure process.
We used function (8) for approximating the data for the trap closing in . The closing develops very quickly; it takes just a fraction of a second. The curve fitting (, ) also shows that the limiting stage of the process is the fluid transfer in the leaf, though it is also very quick due to the small distance between the layers. Parameters of the trap closing were found from curve fitting and presented in . In both experiments (A and B, ) corresponding to the plots in , characteristic time τa is always less than τr. This means that pore opening is relatively fast and it is not a limiting stage.
Our results demonstrate the role of electrical, biochemical and mechanical events leading to the fast trap closure induced by mechanical or electrical stimulation. We developed a new hydroelastic model of the trap mechanism. The novel charge-injection stimulation method presented in this paper provides insight into mechanisms of the different steps of signal transduction and response in the plant kingdom.
Materials and Methods
Digital video recorders Sony DCR-HC36 and Canon ZR300 were used to monitor the Venus flytraps and to collect digital images, which were analyzed frame by frame.
All measurements were conducted in the laboratory at a constant room temperature inside a Faraday cage mounted on a vibration-stabilized table. Ag/AgCl electrodes were prepared from Teflon coated silver wires.Citation38,Citation39 Following insertion of the electrodes into lobes and a midrib, the traps closed. We allowed plants to rest until the traps were completely open. The Charge-Injection MethodCitation2 has been used to precisely estimate the amount of electrical energy necessary to cause trap closure. A double pole, double throw (DPDT) switch was used to connect the known capacitor to the voltage source during charging, and then to the plant during plant stimulation.Citation2,Citation4 Since the charge of capacitor Q, connected to the voltage source V, is Q = CV, we can precisely regulate the amount of charge by changing capacitance and applied voltage. We can instantaneously connect the charged capacitor to the plant and induce an evoked response by changing the switch position.
String stimulus.
The mechanical stimulation was performed by using a cotton thread to gently touch one or two of the six trigger hairs inside the upper leaf of the Venus flytrap. The cotton thread was removed before the leaves closed.
Gelatine stimulus.
Plants were fed a 6 mm × 6 mm × 2 mm cube of 4% (w/v) gelatin. This induces closing by stimulating 2 of the 6 trigger hairs of the Venus flytrap.
Plants.
Three hundred bulbs of Dionaea muscipula (Venus flytrap) were purchased for this experimental work from Fly-Trap Farm (Supply, North Carolina) and grown in well drained peat moss in 250 mL plastic pots at 22°C with a 12:12 hr light:dark photoperiod. The soil was treated with distilled water. All experiments were performed on healthy adult specimens.
Abbreviations
P | = | hydrostatic pressure |
E | = | energy |
CAM | = | the total curvature of the layer A |
CAG | = | the Gaussian curvature |
CA0 | = | the spontaneous or intrinsic curvature of the layer A |
κ | = | the elasticity |
x(t) | = | the distance between edges of the trap |
τa | = | characteristic time of the opening kinetics |
τr | = | characteristic time of fluid transfer |
Figures and Tables
Figure 1 The hydroelastic curvature model. (A) Model composition; (B) Variation of the mean and Gaussian curvatures in the process of Venus flytrap closing.
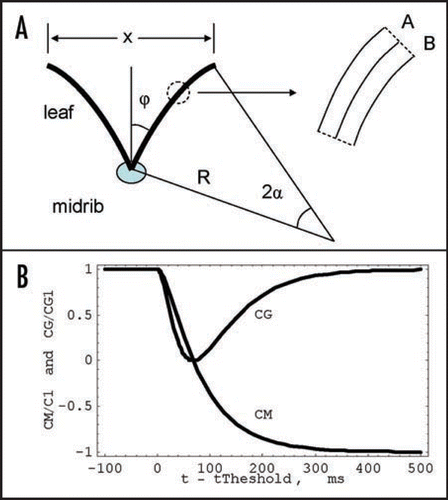
Figure 2 Modeling of the trap closure: (A) Open state; (B) Intermediate state with curvature equal to zero; (C) Closed state. Left column—front view, right column—side view.
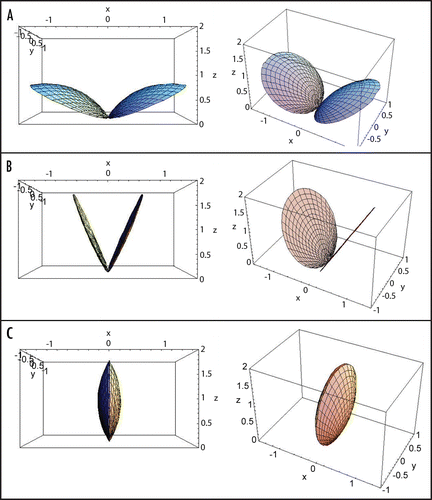
Figure 3 Closing of Venus flytrap by a mechanical stimulation by a cotton thread (A) or gelatin (B) and by electrical 14 µC stimulus (C).
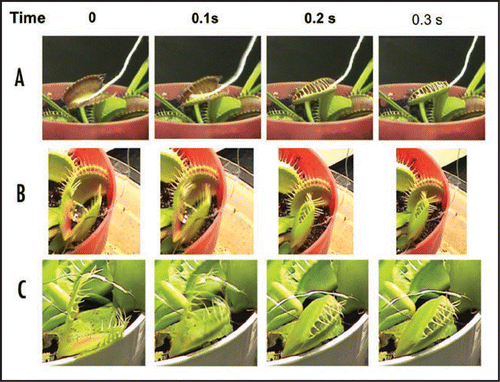
Figure 4 Kinetics of a trap closing stimulated by a cotton thread (▴) or by 14 µC charge (•); x is the distance between the trap margins. Solid lines are theoretical dependencies estimated from equation (8) with parameters from . All these results were reproduced at least ten times. Reproducibility of the initial mechanically silent period with no observable movement of the trap is ±33 ms.
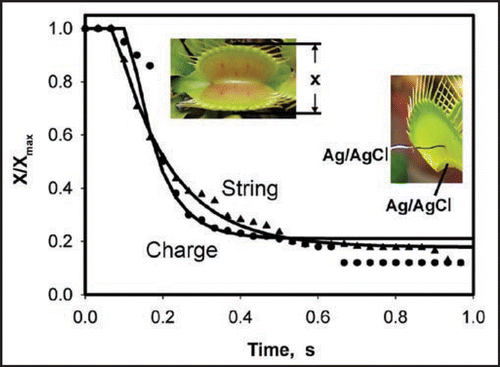
Table 1 Estimated kinetic parameters
References
- Jacobson SL. The effect of ionic environment on the response of the sensory hair of Venus's flytrap. Can J Bot 1974; 52:1293 - 1302
- Volkov AG, Adesina T, Markin VS, Jovanov E. Kinetics and mechanism of Dionaea muscipula trap closing. Plant Physiol 2008; 146:694 - 702
- Burdon-Sanderson J. Note on the electrical phenomena, which accompany stimulation of the leaf of Dionaea muscipula Ellis. Phil Proc R Soc Lond 1873; 21:495 - 496
- Volkov AG, Adesina T, Jovanov E. Closing of Venus flytrap by electrical stimulation of motor cells. Plant Signal Behavior 2007; 2:139 - 145
- Sibaoka T. Physiology of rapid movements in higher plants. Annu Rev Plant Physiol 1969; 20:165 - 184
- Hodick D, Sievers A. The action potential of Dionaea muscipula Ellis. Planta 1988; 174:8 - 18
- Hodick D, Sievers A. The influence of Ca2+ on the action potential in mesophyll cells of Dionaea muscipula Ellis. Protoplasma 1989; 133:83 - 84
- Stuhlman O, Darden E. The action potential obtained from Venus's flytrap. Science 1950; 111:491 - 492
- Brown WH. The mechanism of movement and the duration of the effect of stimulation in the leaves of Dionaea. Amer J Bot 1916; 3:68 - 90
- Benolken RM, Jacobson SL. Response properties of a sensory hair excised from Venus's flytrap. J Gen Physiol 1970; 56:64 - 82
- DiPalma JR, McMichael R, DiPalma M. Touch receptor of Venus flytrap, Dionaea muscipula. Science 1966; 152:539 - 540
- Darwin C. Insectivorous Plants 1875; London Murray
- Lloyd FE. The Carnivorous Plants 1942; New York Ronald
- Jacobson SL. Receptor response in Venus's flytrap. J General Physiol 1965; 49:117 - 129
- Krol E, Dziubinska H, Stolarz M, Trebacz K. Effects of ion channel inhibitors on cold- and electrically-induced action potentials in Dionaea muscipula. Biol. Plantarum 2006; 50:411 - 416
- Jaffe MJ. The role of ATP in mechanically stimulated rapid closure of the Venus's flytrap. Plant Physiol 1973; 51:17 - 18
- Rea PA. The dynamics of H+ efflux from the trap lobes of Dionaea muscipula Ellis (Venus's flytrap). Plant Cell Environm 1983; 6:125 - 134
- Williams SE, Bennet AB. Leaf closure in the Venus flytrap: an acid growth response. Science 1982; 218:1120 - 1121
- Forterre Y, Skothelm JM, Dumals J, Mahadevan L. How the Venus flytrap snaps. Nature 2005; 433:421 - 425
- Hill BS, Findlay GP. The power of movement in plants: the role of osmotic machines. Q Rev Biophys 1981; 14:173 - 222
- Nelson DL, Cox MM. Lehninger principles of biochemistry 2005; 4th Edition New York Freeman 58 - 59
- Bobji MS. Springing the trap. J Biosci 2005; 30:143 - 146
- Fagerberg WR, Allain D. A quantitative study of tissue dynamics during closure in the traps of Venus's flytrap Dionaea muscipula Ellis. Amer J Bot 1991; 78:647 - 657
- Fagerberg WR, Howe DG. A quantitative study of tissue dynamics in Venus's flytrap Dionaea muscipula (Droseraceae) II. Trap reopening. Amer J Bot 1996; 83:836 - 842
- Mozingo HN, Klein P, Zeevi Y, Lewis ER. Venus's flytrap observations by scanning electron microscopy. Amer J Bot 1970; 57:593 - 598
- Beilby MJ, Bisson MA, Shepherd VA. Volkov AG. Electrophysiology of turgor regulation in charophyte cells. Plant electrophysiology—theory & methods 2006; Berlin Springer 375 - 406
- Shimmen T. Volkov AG. Electrophysiology in mechanosensing and wounding response. Plant electrophysiology—theory & methods 2006; Berlin Springer 319 - 339
- Zonia L, Munnik T. Life under pressure: hydrostatic pressure in cell growth and function. Trends Plant Sci 2007; 12:90 - 97
- De Candolle CP. Sur la structure et les mouvements des feuilles du Dionaea muscipula. Arch Sci Phys Nat 1876; 55:400 - 431
- Munk H. Die electrischen und Bewegungserscheinungen am Blatte der Dionaeae muscipula. Arch Anat Physiol Wiss Med 1876; 203 - 230
- Sheetz MP, Singer SJ. Biological membranes as bilayer couples. A molecular mechanism of drug-erythrocyte interactions. Proc Natl Acad Sci USA 1974; 71:4457 - 4461
- Lim HWG, Wortis M, Mukhopadhyay R. Stomatocyte-discocyte-echinocyte sequence of the human red blood cell: Evidence for the bilayer-couple hypothesis from membrane mechanics. Proc Natl Acad Sci USA 2002; 99:16766 - 16769
- Qi Z, Chi S, Su X, Naruse K, Sokabe M. Activation of a mechanosensitive BK channel by membrane stress created with amphipaths. Molecular Membrane Biol 2005; 22:519 - 527
- Markin VS, Albanesi JP. Membrane fusion: stalk model revisited. Biophys J 2002; 82:693 - 712
- Volkov AG, Deamer DW, Tanelian DL, Markin VS. Liquid interfaces in chemistry and biology 1998; New York Wiley
- Tamiya T, Miyazaki T, Ishikawa H, Iriguchi N, Maki T, Matsumoto JJ, Tsuchiya T. Movement of water in conjunction with plant movement visualized by NMR imaging. J Biochem 1988; 104:5 - 8
- Detmers FJM, De Groot BL, Mueller EM, Hinton A, Konings IBM, Sze M, Flitsch SL, Grubmueller H, Deen PMT. Quaternary ammonium compounds as water channel blockers: specificity, potency, and site of action. J Biol Chem 2006; 281:14207 - 14214
- Ksenzhek OS, Volkov AG. Plant Energetics 1998; San Diego Academic Press
- Volkov AG. Green plants: Electrochemical interfaces. J Electroanal Chem 2000; 483:150 - 156