Abstract
Inhibition of cell expansion and modification in the accumulation of cuticle components are two important processes that plants adopted to respond to water deficit. In our recent publication in Plant Journal we characterized an Arabidopsis thaliana transcription factor, belonging to the R2R3-MYB family, specifically expressed in response to water deficit, involved in the regulation of these two processes. The over-expression of this gene causes both the reduction of mRNA level of some genes involved in cell expansion and the accumulation of transcript of genes with a putative role in cuticle synthesis and deposition. In this addendum we propose a model for the function of this transcription factor as an integrator of the pathway that induces cuticle components synthesis and deposition and the process that blocks cell expansion in response to water stress.
One of the first responses to water deficit in most vascular plants is the inhibition of growth which allows plants to reduce their transpiration rates and to avoid metabolic and cellular damage caused by low water potentials.Citation1 Recently in an analysis of different Arabidopsis accessions, it was demonstrated that both cell number and cell area were significantly reduced below a threshold of soil water content.Citation2 The reduction of cell expansion depends on a decrease in cell wall extensibility.Citation3,Citation4 Some lines of evidence support the idea that expansins and xyloglucan endotransglucosylases/hydrolases (XTH) play an important role as wall-loosening proteins in the process of cell expansion (reviewed in ref. Citation5).
Another well described response to water deficit is an increase in wax deposition.Citation6,Citation7 Interestingly stress-resistant plants often have thicker waxes than those that are susceptible to stress. In many species exposure to water deficit and salinity results in increase in wax quantity and modification in the composition of the cuticle; these two aspects cooperate to reduce cuticular transpiration (reviewed in refs. Citation8 and Citation9 ). However, this is not always the case, as other studies showed that the amount of wax had no direct effect on transpiration rate (reviewed in refs. Citation8 and Citation9).
In our recent publication in The Plant Journal we characterised the Arabidopsis AtMYB41 gene, coding for an R2R3-MYB transcription factor.Citation10 This gene is not expressed in normal growth conditions in all the organs analysed, but its expression is significantly induced by different stimuli, particularly by drought stress, ABA and salt treatments. Plants overexpressing AtMYB41 (35S::AtMYB41) showed a pleiotropic phenotype similar to that described for many Arabidopsis cuticle mutants (reviewed in ref. Citation11), including a dwarf stature (resulting from smaller cell size rather than from a decrease in cell number), abnormalities in epidermal cell morphology, decreased fertility. Most important, these plants showed higher surface permeability, as demonstrated by higher water loss and transpiration rates during drought, by the accelerated leaching of chlorophyll from leaves immersed in ethanol and by the higher permeability to toluidine blue staining, the last two assays being the most direct means of screening for cuticular mutants.Citation12,Citation13
From a genome-wide expression analysis of 35S::AtMYB41 and wild-type plants, we identified, among the genes that were upregulated in the overexpression lines, a number of genes, coding for proteins putatively involved in the biosynthesis and deposition of cuticle, like GDSL motif lipases/hydrolases, peroxidases, lipid transfer proteins (LTPs), an ABC transporter. Conversely, among the genes that were downregulated in 35S::AtMYB41 plants, we identified some genes involved in wall loosening, during cell expansion, coding for expansins (AtEXP5) and xyloglucan endotransglucosylases/hydrolases (XTH7 and XTH8). Interestingly genes positively regulated in the transgenic plants were also expressed at high level in response to ABA and to osmotic and salt stress, as AtMYB41. The same stress conditions repressed the expression of genes negatively regulated in 35S::AtMYB41 plants. Taken together, the phenotypic and molecular data presented in our study suggest a dual role for AtMYB41 both as a negative regulator of cell expansion in response to water deficit and as a positive regulator of cuticle synthesis and deposition. In this addendum we propose a model describing AtMYB41 function as a key regulator in the integration of these two responses to water stress (). To our knowledge this is the first transcription factor with such a dual role and our hypothesis is that it acts at a very early step in plant response to stress. Indeed the two processes follow very different pathways and no other convergence points between them have been highlighted so far.
As summarised in under normal growth conditions genes involved in cell expansion are expressed at high level because their function is required to sustain plant growth and development, while their expression is downregulated when plants have to respond to water stress to limit plant transpiration. Therefore it is expected that a negative transcriptional regulator of these genes should not be expressed in normal growth conditions but in response to water stress, as we observed for AtMYB41. On the other hand, if the expression of such a negative regulator is under the control of a constitutive promoter, it should be active also when cell expansion should take place to promote growth and development of plants and the risk is to block this normal process. This is in fact what happens in 35S::AtMYB41 plants that are dwarf, because their cells are smaller than the wild-type ones.
Conversely genes involved in the synthesis and deposition of some cuticle components are expressed at low level under normal growth conditions, while their expression is upregulated in response to osmotic stress. The expression of a positive regulator of this pathway should follow the same pattern of its targets. Again, this is what we observed for AtMYB41. When this positive regulator is overexpressed, the cuticle components described are synthesised at high level also in normal conditions and it causes an incorrect balance among different molecules involved in global cuticle composition that results compromised.
In our model, transgenic plants always mimic a water stress response, but the constitutive expression of AtMYB41 has dramatic negative consequences on plant performances. The use of an inducible promoter to express AtMYB41 in transgenic plants and the availability of a knock-out mutant in this gene will help the future confirmation of our hypothesis.
Figures and Tables
Figure 1 (A) A schematic representation of a model for the role of AtMYB41 as a negative regulator of genes involved in cell expansion and as positive regulator of genes involved in cuticle synthesis and deposition. (B) Expression level of different genes is shown in wild-type and in 35S::AtMYB41 plants in normal growth conditions and in response to water stress. “++” corresponds to high expression level, “+” to low expression level and “−” to no expression.
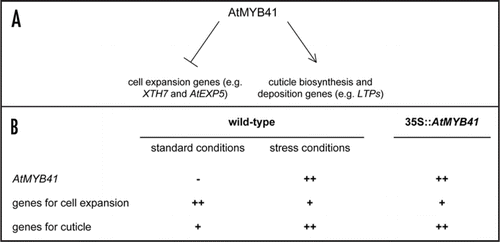
Addendum to:
References
- Westgate ME, Boyer JS. Osmotic adjustment and the inhibition of leaf, root, stem and silk growth at low water potentials in maize. Planta 1985; 164:540 - 549
- Aguirrezabal L, Bouchier Combaud S, Radziejwoski A, Dauzat M, Cookson SJ, Granier C. Plasticity to soil water deficit in Arabidopsis thaliana: dissection of leaf development into underlying growth dynamic and cellular variables reveals invisible phenotypes. Plant Cell Environ 2006; 29:2216 - 2227
- Lu ZJ, Neumann PM. Water-stressed maize, barley and rice seedlings show species diversity in mechanisms of leaf growth inhibition. J Exp Bot 1998; 49:1945 - 1952
- Munns R, Passioura JB, Guo JM, Chazen O, Cramer GR. Water relations and leaf expansion: importance of time scale. J Exp Bot 2000; 51:1495 - 1504
- Cosgrove DJ. Growth of the plant cell wall. Nat Rev Mol Cell Biol 2005; 6:850 - 861
- Bengston C, Larsson S, Liljenberg C. Effect of water stress on cuticular transpiration rate and amount and composition of epicuticular wax in seedlings of six oat varieties. Physiol Plant 1978; 44:319 - 324
- Premachandra GS, Saneoka H, Kanaya M, Ogata S. Cell membrane stability and leaf surface wax content as affected by increasing water deficits in maize. J Exp Bot 1991; 42:167 - 171
- Jenks MA, Eigenbrodeb SD, Lemieuxc B. Somerville CR, Meyerowitz EM. Cuticular waxes of Arabidopsis. The Arabidopsis Book 2002; Rockville, MD American Society of Plant Biologists http://www.aspb.org/publications/arabidopsis/
- Shepherd T, Griffiths DW. The effects of stress on plant cuticular waxes. New Phytol 2006; 171:469 - 499
- Cominelli E, Sala T, Calvi D, Gusmaroli G, Tonelli C. Overexpression of the Arabidopsis AtMYB41 gene alters cell expansion and leaf surface permeability. Plant J 2008; 53:53 - 64
- Nawrath C. Unraveling the complex network of cuticular structure and function. Curr Opin Plant Biol 2006; 9:281 - 287
- Tanaka T, Tanaka H, Machida C, Watanabe M, Machida Y. A new method for rapid visualization of defects in leaf cuticle reveals five intrinsic patterns of surface defects in Arabidopsis. Plant J 2004; 37:139 - 146
- Yephremov A, Schreiber L. The dark side of the cell wall: molecular genetics of plant cuticle. Plant Biosyst 2005; 139:74 - 79