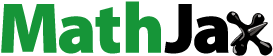
Abstract
Many chemicals are critical for plant growth and development and play an important role in integrating various stress signals and controlling downstream stress responses by modulating gene expression machinery and regulating various transporters/pumps and biochemical reactions. These chemicals include calcium (Ca2+), cyclic nucleotides, polyphosphoinositides, nitric oxide (NO), sugars, abscisic acid (ABA), jasmonates (JA), salicylic acid (SA) and polyamines. Ca2+ is one of the very important ubiquitous second messengers in signal transduction pathways and usually its concentration increases in response to the stimuli including stress signals. Many Ca2+ sensors detect the Ca2+ signals and direct them to downstream signaling pathways by binding and activating diverse targets. cAMP or cGMP protects the cell with ion toxicity. Phosphoinositides are known to be involved both in transmission of signal across the plasma membrane and in intracellular signaling. NO activates various defense genes and acts as a developmental regulator in plants. Sugars affect the expression of many genes involved in photosynthesis, glycolysis, nitrogen metabolism, sucrose and starch metabolism, defense mechanisms and cell cycle regulation. ABA, JA, SA and polyamines are also involved in many stress responses. Cross-talk between these chemical signaling pathways is very common in plant responses to abiotic and bitotic factors. In this article we have described the role of these chemicals in initiating signaling under stress conditions mainly the abiotic stress.
Introduction
Under unfavourable environmental condtions plant growth and development is severely affected which impede the productivity and thus prevent them from reaching their full genetic potential. Indeed, abiotic stresses are the primary cause leading to worldwide crop loss and reducing the average yield for most crops by more than 50%.Citation1 In many plants in response to these stresses various genes gets upregulated resulting in altered metabolic functions, which can mitigate the effect of stress and lead to plant adaptation. Plant responds to stresses as individual cells and synergistically as a whole organism. Stress signal is first perceived by the receptors present on the membrane of the plant cells. Following this the signal information is transduced downstream resulting in the activation of various stress responsive genes (). The products of these stress genes ultimately lead to stress tolerance response or plant adaptation and help the plant to survive and surpass the unfavorable conditions.Citation2–Citation6 The response could also lead to growth inhibition or cell death, which will depend upon how many and which kinds of genes are up or down regulated in response to the stress (). Overall, the stress response is a coordinated action of many genes encoding signaling proteins/factors, including protein modifiers (methylation, ubiquitination, glycosylation, etc.,), adaptors and scaffolds.Citation7
Various chemicals are known to be involved in signal transduction pathways. During the last couple of decades a number of second messengers and hormones that are altered in response to stresses have been identified. Among these the role of calcium and ABA have been well studied and have been reviewed earlier.Citation8,Citation9 In addition to these, in recent years, the role of cyclic nucleotides, polyphosphoinositides, NO, sugars, JA and SA and other hormones have been elucidated. In this review we have briefly covered the recent work on the role of all these chemicals and their downstream signaling components especially under abiotic stress conditions.
Calcium Signaling
Calcium (Ca2+) is an essential plant macro-nutrient element that is taken up by roots and delivered to the shoot via the xylem where it regulate many physiological processes.Citation7,Citation8,Citation10–Citation13 The plant cell contains a number of vesicular compartments, which stores Ca2+ that can be released into the cytoplasm when required. Specific channels/pumps regulate the movement of Ca2+ in and out of cells and organelles.Citation7,Citation13 Using various techniques it has been shown that Ca2+ concentrations in apoplasts ranges between 0.1 to 1.0 mM in vacuoles and ∼1 mM in endoplasmic reticulum lumen and these levels can go upto 50 mM. Plastids and mitochondria contain millimolar levels of Ca2+ but much less than endoplasmic reticulum. The cytosolic free Ca2+ concentration is maintained typically at 200 nM.Citation14 However, the Ca2+ content of the cytosol is far higher than this because of the high affinity for Ca2+ to a range of Ca2+ binding proteins.
The changes in the cytoplasmic calcium concentrations following stress perception have been reported. It was in 1996 that Knight et al., showed biphasic changes in calcium levels in response to cold stress. Similarly water stress and heat induced changes in calcium levels were also reported.Citation15–Citation16 The relationship of these changes with gene expression was also indicated in earlier studies.Citation17 Infact it was found that the changes in calcium were cell type specific.Citation18 Some of this and other work on the role of calcium in stress signaling have been reviewed.Citation19,Citation20 Recently, using various activators and inhibitors, it was found that calcium may be involved even in long-term processing if signals in plants in response to abiotic stresses.Citation21 It thus became evident that calcium is one of the principal candidates for functioning as central node in overall ‘signaling web’ and plays important role in providing stress tolerance. Recently its role in salinity tolerance has been reviewed.Citation8,Citation13 High salinity leads to increase cytosolic Ca2+, which initiates the stress signal transduction pathways for stress tolerance.Citation6,Citation7
While the source of calcium resulting in its increase in the cytoplasm after stress has not be very well studied. Ca2+ release can be primarily from extracellular source (apoplastic space) as addition of EGTA or BAPTA was shown in many cases to block calcium effects (). Ca2+ release may also result from activation of PLC (phospholipase C), leading to hydrolysis of PIP2 to IP3 and subsequent release of Ca2+ from intracellular Ca2+ stores. Furthermore, calcium-binding proteins (calcium sensors) can provide an additional level of regulation in the calcium signaling. These sensor proteins recognize and decode the information provided in the calcium signatures,Citation8 relay the information downstream to initiate a phosphorylation cascade leading to regulation of gene expression (). On the functional basis, CaBP can be classified as trigger proteins and buffer proteins. Trigger proteins get active only after binding to Ca2+ and then interact with other proteins in signal transduction pathway and alter their activity. Buffer proteins bind to the elevated level to sequestre Ca2+. Only few CaBP of trigger type, which are involved in stress mediated Ca2+ signaling, have been identified in plants such as calmodulin (CaM), CaM-binding proteins, Ca2+ dependent protein kinases and phosphatases.Citation12
Calmodulin is one of the important CaBP and contains four calcium binding sites (). Upon receiving stimuli, there is an increase in the intracellular Ca2+. The increase in calcium is sensed by various proteins like calmodulin or directly by calcium dependent protein kinases (CDPKs). The CDPKs have a catalytic domain and calcium binding domain like calmodulin (). There are other calcium binding proteins also which can sense calcium concentration. All these proteins can trigger downstream processes, which can elicit specific response (). The response is terminated by OFF mechanisms that restore Ca++ to the resting level. Various physical and chemical signals have been shown to induce mRNA corresponding to CaM and CaM-related genes and in some cases Ca2+ signals, for example generated via wind and cold stimuli, operate through CaM gene expression (). From various studies it is clear that CaM is a very important protein in Ca2+ signaling and is involved in various process in plants including biotic and abiotic stresses.Citation8,Citation12,Citation22 CaM can also regulate gene expression by binding to specific transcription factors.Citation8,Citation23
Plants possess many unique, putative Ca2+-sensors, including a large family (50 in Arabidopsis) of calmodulin-like proteins termed CMLs. These CaM like proteins differ from the CaM in containing more than 148 amino acid residues and have between one to six EF hand motifs. They possess limited homology to CaM (75% identity with canonical CaM isoforms.Citation12,Citation24 Some of these CMLs have been implicated in Ca2+-based stress response but most remain unstudied. Recently, Venderbeld and Snedden (2007)Citation25 suggested that these CMLs likely play important roles as sensors in Ca2+-mediated developmental and stress response pathways. In Ca2+-mediated signaling many proteins (CaBP) are bound and regulated by CaM and known as CaMBP. In plants some of the examples of CaMBP, which are stimulated by CaM are small nuclear NTPases, glutamate decarboxylase, NAD-kinases, Ca2+-ATPases (ER and tonoplast located), kinesin-like CaMBP, heat-shock proteins and few transcription factors of basic helix-loop-helix family. In Arabidopsis AtCAM3 promoter binds to the leucine zipper family of transcription factor TGA3, while CaM itself acts as an enhancer of TGA 3 binding with its Cis-elements. All these suggests that Ca2+-mediated signaling coupled to gene expression might go via CaM and CaM binding transcription factors and could lead to specificity of the response. It was shown that a Calcium/CaM dependent kinase that was stress regulated phosphorylated a protein that showed binding to stress regulated promoter.Citation20
There are several proteins that bind Ca2+ but do not contain EF-hand motifs. These include, the phospholipase D (PLD), annexins, calreticulin, calnexin and Pistil-expressed Ca2+-binding protein (PCP). The activity of PLD, which cleaves membrane phospholipids into a soluble head group and phosphatidic acid, is regulated by [Ca2+]cyt through a Ca2+/phospholipids binding-site termed as the ‘C2 domain’.Citation26 PLD activity is implicated in cellular responses to ethylene and ABA, α amylase synthesis in aleurone cells, stomatal closure, pathogen responses, leaf senescence and drought tolerance.Citation27 Plants posses several PLD isoforms that differ in their affinity for Ca2+ and their modulation by phosphoinositides, free fatty acids and lysolipids.Citation26 These biochemical modulators of PLD activity are the substrates or products of phospholipase C, which generates IP3, diacylglycerol (DAG), phospholipase A2 and diacylglycerol kinase, both of which are regulated by CaM. It is suggested that [Ca2+]cyt signaling cascades might coordinate the activities of these diverse enzymes to effect specific responses to the environmental stimuli.Citation26
Annexins are a family of proteins in plants and animals that bind phospholipids in a Ca2+ dependent manner and contain four to eight repeats of about 70 amino acids.Citation8 Annexins are encoded by twelve genes in verterbrates and by eight in higher plants. These proteins are involved in organization and function of biological membranes.Citation8 Although exact function of plant annexin is not known, but they are implicated in secretory processes and some have ATPase, peroxidase activities.Citation8 On the basis of existing literature and experimental evidence, Górecka et al., (2007a)Citation28 have proposed that plant annexins may have a role in stress response. Infact, Górecka et al., (2007b)Citation29 have shown that Annexin At1 of Arabidopsis thaliana (AnnAt1), which is one of eight proteins of this family in A. thaliana, play important role in pH-mediated cellular response to environmental stimuli. The ssame group also suggested that the pH-sensitive ion channel activity of AnnAt1 might play a role in intracellular ion homeostasis.
Calnexin (CNX) is a 90 kDa integral memberane protein of the endoplasmic reticulum (ER). It acts as a molecular chaperone that share several functions, including Ca2+ binding, lectin-like activity and recognition of misfolded proteins.Citation30,Citation31 CNX also contributes to the quality control of non-glycosylated polytopic membrane proteins by binding to misfolded or unassembled transmembrane domains. It also plays a role in the degradation of misfolded proteins. Its role in stress has not yet been studied, however it may regulates the endoplasmic reticulum stress response in a manner unique to plants.
Several reports indicate that Ca2+ regulates the transcription of target genes by affecting changes in the phosphorylation status of specific transcription factors. ABA-induced expression of two genes in aurea mutants of tomato, rd-29A, a dessication-induced gene and kin2, a cold-responsive gene are also reported to be Ca2+-regulated. Analysis of transcriptome changes in response to increase in cytosolic calcium revealed that ABRE-related sequences also act as calcium responsive cis-elements.Citation32 It seems therefore that Ca2+, can control many cellular processes in plant cell by interacting with various proteins and kinases, which result in the regulation of expression of various genes. Recently a novel family of transcription factors that bind calmodulin, named CAMTA (calmodulin-binding transcription activators) have been identified and indicated to be involved in stress induced calcium mediated gene expression in Arabidopsis.Citation33,Citation34 The presence of CAMTA has also been shown in rice.Citation35 All CAMTAs have a novel type of sequence specific DNA binding domain and also have IQ motif known to be associated with binding to CaM. CAMTA can also interact with other proteins to and act also as co-activator of transcription.Citation36
In addition to regulating gene expression, another pathway called SOS (salt overly sensitive) pathway, has been implicated in calcium-mediated pathway for salinity stress tolerance.Citation37 As salt stress imposes a major environmental threat to agriculture, understanding the basic physiology and genetics of cell under salt stress is crucial for developing any transgenic strategy. The SOS genes (SOS1–SOS3) were isolated through positional cloning. Since sos mutants are hypersensitive to salt, their characterization resulted in the discovery of a novel pathway, which has helped in our understanding the mechanism of salt stress tolerance in plants. The sos mutants i.e., sos1, sos2 and sos3, could accumulate more proline under salt stress and this accumulation seems to correlate with the extent of damage caused by salt stress.Citation38 All these 3 mutants i.e., sos1, sos2 and sos3 showed a similar spectrum of phenotype indicating that these three SOS genes function in the same or related pathway. The fact that double mutants of these sos genes did not have any additive effect on the salt sensitive phenotype indicated that these genes work in a same pathway leading to salt tolerance.Citation39 However, among the three SOS loci, SOS1 plays the greatest role in imparting salt stress tolerance. Sos1 mutant plants are more sensitive to Na+ and Li+ stress in comparison with sos2 and sos3 mutants.Citation40 Genetic analysis confirmed that SOS1–SOS3 function in a common pathway of salt tolerance. This pathway also emphasizes the significance of Ca2+ signal in reinstating cellular ion homeostasis. SOS3, a Ca2+ sensor, transduces the signal downstream after activating and interacting with SOS2 protein kinase. This SOS3-SOS2 complex activates the Na+/H+ antiporter activity of SOS1 thereby reestablish cellular ion homeostasis. Recently, SOS4 and SOS5 have also been characterized.Citation37 SOS4 encodes a pyridoxal (PL) kinase that is involved in the biosynthesis of pyridoxal-5-phosphate (PLP), an active form of vitamin B6. SOS5 has been shown to be a putative cell surface adhesion protein that is required for normal cell expansion. Under salt stress, the normal growth and expansion of a plant cell becomes even more important and SOS5 helps in the maintenance of cell wall integrity and architecture.
In addition to the nature of calcium signal, discussed earlier, the specificity of Ca2+ signals will also depend on the concentration and activity of these proteins. The specificity of the response will also depend upon the source of Ca2+. For example in response to elicitor, GA and water stress, the source of Ca2+ is from extracellular or apoplastic while in response to wind and touch the source is from intracellular stores. However, for cold shock induced responses the source of Ca2+ could be both extracellular stores and intracellular which is IP3-mediated.
The multifunctional role of Ca2+ suggests that it participates in many signaling pathways in the plant cell. In fact an indirect increase in [Ca2+]cyt in plant cell is mediated by the increase in intracellular concentrations of other second messengers such as cGMP, cAMP, IP3 etc., in response to stimuli. It is clear now that the elevation of Ca2+ concentration in cytoplasm is a key event in the plant cell for transduction of various signals to a biological effect. This suggests that Ca2+ is a communication point in the cytoplasm for the crosstalk between different signal transduction pathways and finally helps interchanging the information.
Signaling via Cyclic Nucleotides
cAMP and cGMP are ubiquitous second messengers and play very important role in cell signaling. In fact cAMP was the first intracellular messenger to be discovered. The levels of cAMP and cGMP in the cell are determined by the activities of cyclases (which catalyze their synthesis) and phosphodieserase (PDE; which hydrolzye them), as shown below:
There have been a number of reports showing the presence of cyclic AMP in plants. A cDNA encoding adenylate cyclase was also reported from ax1-141 tobacco line, which also suggested the existence of cAMP signaling in plant. Its role has been suggested in flowering, in auxin-triggered cell division in higher plants, in the cell cycle control of tobacco cell culture, and in the activation of ion channels that were permeable for Ca2+ and were negatively regulated by CaM.
Kohler et al., (1999)Citation41 have reported a new gene family in Arabidopsis, which share features with cyclic-nucleotide-gated channels from animals and inward-rectifying K+ channels from plants. This gene family comprised of six members of cyclic nucleotide-gated channels, with significant protein sequence homology among them. An extracellular nucleotide PDE from tomato cultured cells has been purified. The purified enzyme has a molecular mass of 70 kDa, a pH optimum of 6.2 and an isoelectric point of 8.1. The enzyme was shown to induce with RNase LE and acid phosphatase upon phosphate starvation of suspension cultured tomato cells. These authors also provided evidence that tomato cyclic nucleotide PDE was an accessory ribonucleolytic activity required for complete degradation of extracellular RNA.
In Arabidopsis, cGMP was shown to modulate the activity of inward rectifying K+ channels KAT1 and AKT1. High salinity stress is a major cause of sodium toxicity in plants. Still not much is known about the mechanisms that underlie Na+ influx. Maathuis and Sanders (2001)Citation42 reported that sodium uptake in Arabidopsis roots is regulated by cyclic nucleotides. The presence of micromolar concentrations of cAMP or cGMP at the cytoplasmic side of the plasma membrane evoked a rapid decrease in channel open probability. The short-term unidirectional Na+ ions influx was also reduced in the presence of cyclic nucleotides. In summary, the data of Maathuis and Sanders (2001)Citation42 suggested that Arabidopsis plants might contain a cyclic nucleotide-based signaling pathway that directly affects Na+ ions transport via voltage independent channels. In a later study, Maathuis (2006)Citation43 studied transcriptome following treatment with membrane permeable cGMP and found changes in many transcripts including those involved in defence and cGMP also modulated Na+ and K+ fluxes.
Phosphoinositide Signaling
Phosphoinositides are key components of the inositol 1,4,5-trisphosphate/diacylglycerol-based signaling pathway in animal cells. In plant cells different phosphoinositide isomers have also been identified.Citation44 Phosphoinositides are the inositol-containing phospholipids of plasma membrane of plant cells and are known to be involved both in transmission of signal across the plasma membrane and in intracellular signaling. The inositol head group can be phosphorylated at several different positions, giving rise to a number of multiple phosphorylated species like phosphatidylinositol (PI), PI-4-monophosphate (PI(4)P or PIP) and PI 4, 5-biphosphate (PI(4,5) P2 or PIP2).Citation45 These polyphosphoinositides are continuously being formed in the inner leaflet of the plasma membrane and are acted upon by set of specific kinases and phosphatases and thereby are kept in a state of constant turnover. One such kinase of significance is PI3 kinase (PI3Kase). Phospholipid metabolism is involved in hyperosmotic-stress responses in plants.Citation46
In plants phospholipases are required for signal transduction in many events like during seed germination, in auxin-stimulated cell elongation, oil biosynthesis during embryo maturation, for membrane reorganization/lipid recycling, and in light mediated processes.Citation47,Citation48 The action of phospholipases can generate three important signal molecules IP3, DAG and PA (). PLD catalyzes the hydrolysis of the phosphodiester bond between the phosphate and the polar group (inositol, serine, glycerol, choline, ethanolamine) and can produce phosphatidic acid. PI specific PLC uses PIP2 as a substrate to release DAG and inositol triphosphate (IP3). Overall, phospholipases they play an important role in plant growth and development including their role in stress tolerance.Citation47,Citation48 Their catalytic properties are often controlled by phosphorylation, interaction with other proteins (e.g., G proteins), as well as interactions with other lipids.Citation48 The signaling through PI3K, phosphotidic acid, IP3 and DAG are described below.
Phosphatidylinositol 3-kinases (PI3Ks).
The PI3K family of enzymes phosphorylates D-3 position of inositol ring (i.e., 3′ hydroxyl group) of PI, PIP and PIP2 resulting in the formation of PI-3-phospate (PI-3P), PI-3,4-biphosphate (PI-3,4-P2) and PI-3,4,5-triphosphate (PI-3,4,5-P3) respectively.Citation49 Both PI(3,4) P2 and PI(3,4,5)P3 are thought to be second messenger molecules that interact with target proteins to change their activity within the cell. In animal systems P13K has been shown to be a component of a many tyrosine kinase complexes that transduce growth factor signal(s) for cell division. The PI3-Ks are now known to be involved in a plethora of cellular processes, ranging from mitogenesis, membrane trafficking and ruffling, to glucose uptake, oxidative burst responses, chemotaxis and apoptosis. PI3K is composed of a catalytic (110 kDa) and regulatory (85 kDa) subunit.Citation49
In plant, this is known to be present in Arabidopsis, soybean and carrot.Citation50–Citation52 The Arabidopsis PI3K (AtVPS34) was shown to be involved in plant growth and development.Citation50 In soybean, PI3K was reported to be induced during nodule organogenesis and was shown to be associated with membrane proliferation.Citation51 In carrot, PI3K activity was associated with the cytoskeleton.Citation52 In carrot suspension cells, PI3K has been shown to be localized on the transcription sites present in the nucleus and nucleolus.Citation52 Stomatal guard cells have been reported to contain PI3P and PI3K activity. Guard cells generate reactive oxygen species (ROS) in response to abscisic acid (ABA), which leads to stomatal closing. The upstream steps of the ABA-induced ROS generation pathway are not well studied. Park et al., (2003)Citation53 reported that PI3K inhibitors wortmannin or LY294002 inhibited ABA-induced ROS generation and stomatal closing, suggested the role of this in ABA-induced ROS generation in guard cells. It has been also reported that activation of PI3K and its product PI3P are required for auxin-induced production of ROS and root gravitropism.Citation54 Recently, Leshem et al., (2007)Citation55 have shown that salt stress responses, such as increased plasma membrane endocytosis and the intracellular production of ROS, were coordinated by phospholipid-regulated signaling pathways. These authors observed that induction of PI3K-mediated endocytosis by salt stress leads to intracellular production of ROS and salt tolerance, which suggested that ROS act in the signal transduction of the salt tolerance response.Citation55
Phosphatidic acid (PA).
PA and its phosphorylated derivative diacyl-glycerol pyrophosphate (DAG-PP) are lipid molecules that have been implicated in plant stress signaling.Citation56 It is one of the second messenger generated by activation of two different signaling pathways, those of PLC and PLD.Citation6,Citation48 PLC hydrolyses PIP2 into IP3 and DAG. IP3 diffuses into the cytosol, where it releases Ca2+ from intracellular stores, whereas DAG remains in the membrane, where it is phosphorylated to PA by DAG kinase.Citation48 However, PLD produces PA directly by hydrolysing structural phospholipids such as phosphatidylcholine.Citation57 DAG kinase was reported to be the dominant producer of PA that was subsequently metabolized to DAG-PP.Citation48 Since PA formation was reported to correlated with the high turnover of polyphosphoinositides, it was suggested that elicitor treatment activates PLC to produce DAG, which in turn acts as substrate for DAG kinase.Citation48
In plants, PA level increases within minutes of wide variety of stress treatments including ethylene, wounding, osmotic stress, oxidative stress, drought, pathogen elicitors and abscisic acid.Citation53 The enhanced PA signaling in plants is attenuated by phosphorylating it to DAG-PP via a PA kinase. Whether the formation of DAG-PP is just a mechanism for attenuating PA levels or whether DAG-PP also functions as a signaling molecule remains to be seen. Several downstream targets of PA have been identified, including protein kinases and ion channels.Citation58 In general PA does not act by itself but it cross talks with other pathways, such as those controlled by Ca2+, G-proteins, protein kinases, MAP kinases and reactive oxygen species. Recently, it was found that PA binds to CTR1, which is a negative regulator of ethylene signaling pathway.Citation59 In addition to PA, a PA metabolite, DAG pyrophosphate (DGPP), has been found in plants and this species is absent in higher animals. Its levels are very low under normal condtions but increase in response to stress. It is broken down by DGPP phosphatase.Citation60
IP3 and DAG.
As mentioned above, in response to a stimulas the PLC gets activated and hydrolyzes PIP2 to release two important second messengers viz IP3 (inositol 1,4,5, trisphosphate) and DAG.Citation8,Citation48 IP3 is known in animal systems to release calcium from endoplasmic reticulum while in plants it has been shown that vacuole is the major store house for calcium.Citation8,Citation48 It has been shown, using TLC and gas chromatography, and comparing constitutive and stress inducible poyphospohinositides, that there was loss in unsaturated phosphatidylinositol which are also channeled towards IP3 production.Citation61 IP3 has also been shown to play important role in abiotic stress signaling. Pical et al., (1999)Citation44 have shown that phosphatidylinositol 4,5-bisphosphate, diacylglycerol pyrophosphate and phosphatidylcholine in Arabidopsis thaliana cells increases rapidly in response to salinity and hyperosmotic stress. Drobak and Watkins (2000)Citation46 found that salts and osmotic agents increased the levels of IP3 by 15-fold as one of the initial response. Takahashi et al., (2001)Citation62 also reported that hyperosmotic stress induced a rapid and transient increase in inositol IP3 independent of abscisic acid in Arabidopsis cell culture.
Though DAG has been shown to increase in response to exogenous stimuli, the downstream signal transduction pathways have not been well characterized in plants. In animal systems it activates protein kinases of C type. In plants there are only a few reports on the existence of PKC and its role has been shown in light and in stress responses.Citation63 As mentioned above DAG could also get converted to PA, which can then act as a signal molecule.
Proline accumulation is reported to occur in various plant organisms in response to environmental stresses. Recently role of PLC in proline accumulation was shown by the use of U73122, which inhibits PLC activity and it also inhibited the enzyme P5CS that is involved in proline accumuation.Citation64 These studies have indicated IP3 mediated calcium release may be important for stress mediated proline accumulation. Moreover, the utilization of diacylglycerol kinase and InsP(3)-gated calcium release receptor inhibitors suggested that InsP(3) or its derivatives are essential for proline accumulation upon salt stress, involving calcium as a second messenger in ionic stress signaling.Citation64,Citation65 These results also demonstrated the specific involvement of lipid signaling pathway to discriminate between ionic and nonionic stresses.Citation64 Recently, Tang et al., (2007)Citation65 reported that in Arabidopsis thaliana, cytosolic Ca2+ oscillations are synchronized to extracellular Ca2+ concentration oscillations largely through the Ca2+-sensing receptor CAS. CAS regulates concentrations of IP3, which in turn directs release of Ca2+ from internal stores. The oscillating amplitudes of cytosolic Ca2+ and extracellular Ca2+ are controlled by soil Ca2+ concentrations and transpiration rates.
Nitric Oxide Signaling
In 1867, amyl nitrate was used clinically by Brunton for the treatment of angina pectoris. Today we know that amyl nitrate is a compound that liberates nitric oxide (NO).Citation66 NO is an intra- and extracellular messenger that mediates diverse signaling pathways in target cells and is known to play important role in many physiological processes. An extensive work in the animal systems has led to the acceptance of NO as a component of second messenger cascades of hormone and physiological signals.Citation66 NO is synthesized as one of the product of a reaction in which L-arginine is metabolized to L-citrulline by nitric oxide synthase as shown in . In this reaction NADPH is used as an electron donor and O2 as co-substrate. The cofactors FMN, FADH and tetrahydopterin are also required. There are at least three isoforms of nitric oxide synthase (NOS). Two of these are constitutive and one is inducible (iNOS or NOSII). Of the two constitutive forms is classified as neuronal (n NOS or NOSI) and the other as endothelial (eNOS or NOSIII). The constitutive NOS isoforms require calcium and calmodulin for their activity. The NOSI is a 160 kDa, NOSII a 135 kDa and NOSIII a 135–140 kDa protein.Citation66 From the N-terminal end lie the domains for binding to arginine, H4B, heme and calmodulin and from the C-terminal end binding sites for NADPH, FMN and FAD are present. As active NOS is a homodimer. Nitric oxide has been shown to modulate its action via stimulating proteins like ADP ribosyl transferase, cycloxygenases and more importantly via soluble guanylate cyclases. It can also inhibit the activity of lipoxygenase, H+ ATPase, ribonuceotide reductase, aconitase etc. In plants the presence and the activity of NOS has been reported in many systems by western blot analysis using antibodies against animal NOS and also by checking the conversion of labeled arginine to citrulline.Citation66
NO is soluble in both aqueous and lipid media, but is relatively reactive and easily oxidized to other nitrogen oxides. It reacts with superoxide to form peroxynitrite, with other cellular components such as transition metals and haem-containing proteins and with thiol groups to form S-nitrosothiols. Thus, diffusion of NO within the plant may be relatively restricted. It is possible that NO is transported as chemical precursors such as nitrite or as nitrosothiols that might function as NO reservoirs. Studies have also revealed a role for NO as signaling molecule in plant, as it activates various defense genes and acts as developmental regulator.Citation66 It was however been shown that in plants nitrate reductase may be involved in generating NO by catalyzing NAD(P)H dependent reduction of nitrate to NO.Citation66 Infact this pathway of NO generation was found to be involved in ABA-induced stomatal closure (). In detailed studies on pathogen signaling, it was found that after pathogen attack the machinery for NO synthesis, NOS or other NO-generating enzymes may be activated thus leading to an increase in NO which inturn could activate guanylate cyclase leading to an increase in cGMP. Details of downstream signaling targets, however, are not very well worked out.
As a developmental regulator, NO promotes germination, leaf extension and root growth, and delays leaf senescence and fruit maturation. Moreover, NO acts as a key signal in plant resistance to incompatible pathogens by triggering resistance-associated hypersensitive cell death. In addition, NO activates the expression of several defense genes (e.g., pathogenesis-related genes, phenylalanine ammonialyase, chalcone synthase) and could play a role in pathways leading to systemic acquired resistance.Citation67 A novel role for NO in the regulation of lateral root development in tomato has been reported which probably operates in the auxin signaling transduction pathway.Citation68 NO has been associated with plant defense responses during microbial attack, and with induction and/or regulation of programmed cell death.Citation69 The expression of genes in response to NO has also been shown to mediate via SA and JA signaling pathway.Citation70
Recently, Ali et al., (2007)Citation71 showed that Arabidopsis thaliana cyclic nucleotide gated channel-2 (CNGC2/DND1) conducts Ca2+ into cells and provide a model linking this Ca2+ current to downstream NO production. Plants without functional CNGC2 lack this cell membrane Ca2+ current and do not display hypersensitive response (HR); providing the mutant with NO complements this phenotype. The bacterial pathogen-associated molecular pattern elicitor lipopolysaccharide activates a CNGC Ca2+ current, which may be linked to NO generation due to buildup of cytosolic Ca2+/calmodulin.Citation67 Recently, it was found that NO is required for the production of lipid second messenger, PA, via activation of PLC and DAG in tomato cell cultures treated with xylanase, a fungal elicitor. PA was required for xylanase induced ROS production.Citation72 These results indicated that PLC/DAG kinase-derived PA represents a novel downstream component of NO signaling cascade during plant defense. This also shows cross talk between two different signaling pathways during plant defense. Graziano and Lamattina, (2007)Citation73 recently reported that NO accumulation is required for molecular and physiological responses to iron deficiency in tomato roots. NO action in plants also seems to be closely linked to SA and hence it could mediate many processes via SA signaling pathway. Infact biochemical, molecular and genetic studies reveal an interaction of NO, ROS, Ca2+, SA, JA and ethylene in many biotic stress responses and also in developing cross tolerance in plants.Citation69–Citation72
NO is also known to play a role in regulation of stomatal closure. Little is known about the signalling events occurring downstream of NO. Previous studies have shown that NO modulates cytosolic calcium concentration and the activation of plasma membrane ion channels.Citation69–Citation73 There is some evidence to show that calcium and MAPK can mediate NO signaling.Citation74 Zhang et al., (2007)Citation75 have found that NO and H2O2 regulate K/Na ratio in callus of Populus eupharatica under salt stress. Recently, Distefano et al., (2008)Citation76 have provided a evidence that supported the involvement of the lipid second messenger PA in NO signalling during stomatal closure.
Sugar Signaling
The production, utilization, mobilization and allocation of photosynthate in various tissues at different stages of development are highly regulated as these processes are required to meet the carbon and energy demands of the system. Sugars are essential to plant growth and metabolism, both as energy source and as structural components. Sugars such as sucrose serve dual functions as transported carbohydrates in vascular plants and as signal molecules that regulate gene expression and plant development.Citation77 Sugars affect the expression of many genes involved in photosynthesis, glycolysis, nitrogen metabolism, sucrose and starch metabolism, defence mechanisms and cell cycle regulation and therefore studies have been undertaken to find out sugar sensing and signal transduction pathways.Citation78 In many systems hexokinase 1 (HXK1) has been implicated to be an evolutionarily conserved glucose sensor that integrates nutrient and hormone signals to govern gene expression and plant growth in response to environmental cues.Citation79 However, the molecular mechanism through which HXK1 controls the expression of genes encoding proteins involved in photosynthesis is not well understood. Arabidopsis plants shows that there may be broadly two glucose signal transduction pathways: hexokinase dependent, and hexokinase independent pathway.Citation78 Recent research demonstrates that a previously unknown HXK1 nuclear complex controls the expression of specific photosynthetic genes, a process that is independent of glucose metabolism but requires two unexpected partners, VHA-B1 and RPT5B,Citation80 which suggested that metabolic enzymes can play unique roles in signal transduction by directly controlling gene expression in the nucleus. In plants the sugar signaling may also cross talk to other signaling pathways.
To understand the molecular mechanisms of sugar responses, Li et al., (2007)Citation81 have reported an Arabidopsis thaliana mutant, high sugar responseCitation8 (hsr8), which showed enhanced sugar-responsive growth and gene expression. These authors observed that light-grown hsr8 plants exhibited increased starch and anthocyanin and reduced chlorophyll content in response to glucose treatment, while dark-grown hsr8 seedlings showed glucose-hypersensitive hypocotyl elongation and development. Their further findings revealed a pathway that signals changes in the cell wall through PRL1 (pleiotropic regulatory locus1) to altered gene expression and sugar-responsive metabolic, growth and developmental changes.Citation81 The level and activity of sucrose synthase (SUS) enzyme was found to be high in sink organs but low in source organs. Recently, Qiu et al., (2007)Citation82 reported that light and metabolic signals regulating SUS protein degradation in maize (Zea mays) leaves during deetiolation. These findings suggested that SUS degradation is important to supply residues for the synthesis of other proteins required for autotrophic metabolism.
Starch is known to be the main carbohydrate store in plants. Regulation of starch metabolism, in particular in response to environmental cues, is of primary importance for carbon and energy flow in plants. Recently, Kempa et al., (2007)Citation83 provided an evidence that MsK4, a novel Medicago sativa Glycogen synthase kinase 3-like kinase, connects stress signalling with carbon metabolism. MsK4 was found to be a plastid-localized protein kinase that is associated with starch granules. High-salt stress rapidly induced the in vivo kinase activity of MsK4. Plants overexpressing MsK4 showed improved tolerance to salt stress, which also accumulated significantly more starch and showed modified carbohydrate content compared with wild-type plants. These important finding suggested that MsK4 is an important regulator that adjusts carbohydrate metabolism to environmental stress.Citation83 Baena-Gonzalez et al., (2007)Citation84 have shown that protein kinases KIN10 and KIN11 are the central points in linking stress, sugar and developmental signals to regulate metabolism, energy balance, growth and survival under stress.
Signaling via Phytohormones and Growth Regulators
Phytohormones are critical for plant growth and development and play an important role in integrating various stress signals and controlling downstream stress responses. It is now well established that the stress-induced gene products are also involved in the generation of regulatory molecules like ABA. However, in recent years regulation and involvement of other hormones like cytokinins,Citation85 auxins,Citation86,Citation87 ethyleneCitation88 and brassinosteroidsCitation89,Citation90 have been indicated. There are indications that volatile organic compounds, which are involved in signaling in plant-insect interactionsCitation91,Citation92 may also be induced by exposure of plants to high light and high temperature conditions.Citation93 Thus in addition those regulators which were thought to be involved in only biotic signaling are now being shown to be involved in abiotic signaling too suggesting cross talk between the abiotic and biotic pathways. In this context here we described the role of ABA, SA, JA and polyamines, whose production under stress can initiate the second round of signaling.
Abscisic acid (ABA).
It is known to be generated as a endogenous messenger during a plant's life cycle to control various physiological processes such as seed dormancy and delays its germination, development of seeds, promotion of stomatal closure, embryo morphogenesis, synthesis of storage proteins and lipids, organ senescence and also defense against pathogens.Citation9,Citation94 Since various stresses induce ABA synthesis, therefore it is now considered as a plant stress hormone.Citation6–Citation9,Citation95 Plants have to adjust ABA levels constantly in responce to changing physiological and environmental conditions. The application of ABA to plant mimics the effect of a stress condition.Citation9 As many abiotic stresses ultimately results in desiccation of the cell and osmotic imbalance, there is an overlap in the expression pattern of stress genes after cold, drought, high salt or ABA application.Citation9 This suggests that various stress signals and ABA share common elements in the signaling pathway and these common elements cross-talk with each other, to maintain cellular homeostasis.Citation9 Various transcription factors are known to regulate the ABA-responsive gene expression.Citation6,Citation9
Main function of ABA seems to be the regulation of plant water balance and osmotic stress tolerance. Several ABA deficient mutants namely aba1, aba2 and aba3 have been reported for Arabidopsis.Citation96 ABA deficient mutants for tobacco, tomato and maize have also been reported.Citation95 Without any stress treatment the growth of these mutants is comparable to wild type plants. Under drought stress, ABA deficient mutants readily wilt and die if the stress persists. Under salt stress also ABA deficient mutants show poor growth.Citation97 In addition, ABA is required for freezing tolerance, which also involves the induction of dehydration tolerance genes.Citation97 Studies suggest that osmotic stress imposed by high salt or drought is transmitted through at least two pathways; one is ABA-dependent and the other ABA-independent. Cold exerts its effects on gene expression largely through an ABA-independent pathway.Citation9 The transcript accumulation of RD29A gene and the Proline accumulation in plants are reported to be regulated in both ABA-dependent and ABA-independent manner.Citation98 Auxin homeostasis is also known to be directly linked to growth regulation with stress through interactions with SA and ABA signals.Citation99 Several studies have demonstrated that ABA, drought, cold and high salt result in rapid increase in calcium levels in plant cells.Citation6–Citation9,Citation95 The salinity stress-induced upregulation of transcript of pea DNA helicase 45 (PDH45) followed ABA-dependent pathway,Citation100 while calcineurin B-like protein (CBL) and CBL-interacting protein kinase (CIPK) from pea followed the ABA-independent pathway.Citation101 Overall, the ABA-dependent pathways are involved essentially in osmotic stress gene expression. The role of ABA in drought tolerance in plant has been repored by Xiong et al., (2006).Citation102 Recently, Cao et al., (2007)Citation103 have reported that ABA and stress signals induce Viviparous1 (Vp1) expression in seed and vegetative tissues of maize. The Vp1 encodes a B3 domain-containing transcription factor that is a key regulator of seed maturation in maize.
It is now clear that to survive environmental stresses plants must respond to ABA. Though lot of work on the involvement and relation ship of this hormone to abiotic stress response, especially drought stress, has been done, and ABA-dependent Cis elements (ABRE) have been reported in many stress responsive genes, the receptor of this hormone was not found till recently. Razem et al., (2006)Citation104 have shown that an RNA binding protein, FCA, which also has role in flowering, is ABA receptor. This work should now reveal how under stress the flowering behaviour is altered in different plants. To obtain more information about ABA signaling network, Zhang et al., (2008)Citation105 carried out a genetic screen about ABA supersensitive mutant (absg1 and absg2) on the basis of an estradiol-inducible activation-tagged Arabidopsis mutants and reported that the disturbance of small RNA pathways enhanced ABA response and multiple stress responses in Arabidopsis. This report provided more physiological evidences that linked small RNA pathway and ABA signaling.
Jasmonates (JA).
Jasmonates are lipid-derived signaling molecules involved in induced systemic resistance, wounding and stress responses as well as in plant growth and development. Many of them alter gene expression positively or negatively in a regulatory network with synergistic and antagonistic effects in relation to other plant hormones such as salicylate, auxin, ethylene and abscisic acid.Citation106 It is reported in tobacco that, wounding causes rapid activation of two mitogen-activated protein kinases (MAPKs), wound-induced protein kinase (WIPK) and salicylic acid (SA)-induced protein kinase (SIPK), and the subsequent accumulation of jasmonic acid. It is found that activation of WIPK is required for the production of wound-induced JA. Recently, Seo et al., (2007)Citation107 suggested that WIPK and SIPK play an important role in JA production in response to wounding, and that they function cooperatively to control SA biosynthesis. In Arabidopsis the systemic immunity uses conserved defense signaling pathways, which is found to mediate by jasmonates.Citation108 These authors suggested that jasmonate signaling mediate long-distance information transmission. Moreover, the systemic transcriptional response shares extraordinary overlap with local herbivory and wounding responses, indicating that jasmonates may be pivotal to an evolutionarily conserved signaling network that decodes multiple abiotic and biotic stress signals.
Recentlt, Walia et al., (2007)Citation109 reported the jasmonic acid-mediated adaptation of barley to salinity stress. The JA-pre-treated salt-stressed plants accumulated low levels of Na+ in the shoot tissue compared with untreated salt-stressed barley plants after several days of exposure to stress. Their study suggested that three JA-regulated genes, arginine decarboxylase, ribulose 1.5-bisphosphate carboxylase/oxygenase (Rubisco) activase and apoplastic invertase are possibly involved in salinity tolerance mediated by JA.
Salicylic acid (SA).
It is also a plant signaling molecule and known to play a critical role in defense responses after pathogen attack. It antagonizes gene induction by the stress signaling molecule JA. Dat et al., (1998)Citation110 reported that SA can induced thermotolerance in mustard seedlings. Borsani et al., (2001)Citation111 showed an evidence for a role of SA in the oxidative damage generated by NaCl and osmotic stress in Arabidopsis seedlings. We have earlier reported that the CBL and CIPK genes from pea were induced in response to SA and wounding.Citation101 Several SA-responsive genes are known to regulate by basic/leucine zipper-type transcription factors of the TGA family. TGA factors interact with NPR1, a central regulator of many SA-induced defense responses including SA/JA antagonism. An ATPase C gene from Pennisetum was found to be upregulated by salicylic acid and its promoter was found to bind nuclear factors to TGA cis elements.Citation112 Recently, Ndamukong et al., (2007)Citation113 reported that glutaredoxin acts as regulatory protein of SA-dependent signaling pathways. Koo et al., (2007)Citation114 reported that overexpression of SA carboxyl methyltransferase reduces SA-mediated pathogen resistance in Arabidopsis thaliana. Their results indicated that in the absence of SA, methyl salicylate alone cannot induce a defense response, yet it serves as an airborne signal for plant-to-plant communication. Recently, Wang et al., (2007)Citation115 reported that SA inhibits pathogen growth in plants through repression of the auxin signaling pathway.
Polyamines.
Polyamines, a group of small aliphatic amines, are reported to play role in plant development. These are also known to accumulate under salt stress conditions in different plant systems, resulting in presumed protective effects, acting as free radical scavengers, stabilizing cellular membranes and maintaining cellular ionic balance under these conditions. Jiménez-Bremont et al., (2007)Citation116 reported that in response to long-term salt stress the levels spermidine and spermine were modulated suggesting their role in salt stress. These authors also suggested that there is a connection between polyamine metabolism, abiotic stress and abscisic acid. Polyamines role in other stress responses has also been shown.Citation117,Citation118 It was found by Yamaguchi et al., (2006)Citation119 that an arabidopsis double knock out plant that cannot produce spermine showed higher sensitivity to high salt. This mutant was also later found to be sensitive to drought and the mutant phenotype could be cured by the addition of spermine but not by spermidine.Citation120 The genes involved in spermine and spermidine synthesis have also been found to be regulated by salinity and ABA.Citation116 By using patch clamp techniques to protoplasts, it was found that polyamines affect K+/Na+ homeostasis.Citation121 This could be one of the mechanism by which polyamines could help in salinity tolerance in plants. Regulation of polyamines biosynthesis has also been reported in plants under drought stress. In cacao, expression pattern of five genes encoding enzymes involved in polyamines biosynthesis were studied in response to drought and correlated with the levels of putresicine, spermine and spermidine.Citation122 In many studies overexpression of these genes has also been shown to confer stress tolerance in plants.Citation118 There is evidence of cross-talk of polyamine with NO. Exogenous addition of polyamine has been shown to induce the production of NO although the mechanism for this is still not understood and needs more experiments.Citation123
Conclusion and Perspectives
The essential feature of plant survival to changing environmental conditions is their ability to continuously monitor fluctuations in light conditions, temperature variations, water and nutrient availability, CO2 levels etc. The sensitivity of the sensors to evaluate the intensity and limits of variation lead to the induction of physiological defense mechanisms. In this review we have dealt with the nature of chemicals which are produced following perception of stress environment and carry the message in the cell to respond either directly of indirectly by regulating the expression of genes leading to altered functions or enhance the capacity of the already existing defense metabolites. These chemicals remain in a state of flux; their levels are increased or decreased according to the demand put on plants by the intensity and the nature of the abiotic stress. Thus abiotic stress signaling is an important area with respect to increase in crops yield under sub-optimal conditions.
During the last few years the information on the nature of chemicals and the signaling pathways that they are involved has been generated. Earlier, it was thought that calcium, phosphoinositides and ABA were the key molecules that participated in abitoic stress signaling. Recently, calcium signaling is also reported to involved in the regulation of plant cell cycle progression in response to abiotic stress.Citation124 However, as it has been described in this review chemicals like SA, JA, NO, which were thought to respond to biotic stresses, have now been implicated in abiotic stresses also. The role of other molecules like simple sugars and polyamines have also been elucidated which has been covered in this review. It seems that there may be many others, which may also be playing the role as signal molecules. Lately, chemicals like glutathione and trehalose-6-phosphate, which are involved as antioxidant and osmolyte, have also been shown to act as signaling molecules and induce the expression of genes.Citation125,Citation126 Genetic analyses have revealed extensive interactions between sugar and plant hormone signaling. Important and complex roles for Snf1-related kinases (SnRKs), extracellular sugar sensors and trehalose metabolism in plant sugar signaling are now also emerging.Citation78
In future more work should be done to find out their exact role. An effort should also be taken up to find out the function of brassinosteroids in more details. Cellular perception of NO may occur through its reaction with biologically active molecules that could function as ‘NO-sensors’. But exactly how NO evolution relates to its bioactivity in planta remains to be established.Citation127 We envisage that more molecules will be discovered that play an important role in signal transduction under abiotic stresses. The overall progress of research on chemical regulated stress responsive genes and their products reflect their central role in plant growth and development under stress conditions. The mechanisms, by which the plants maintain their physiology and development under abiotic stress using different chemical signals at different times and in response to different stress conditions, and cross talk between each of them, still remained to be fully understood. Overall, it is becoming clear that chemicals action enforces a sophisticated regulation at all levels.
Figures and Tables
Figure 1 Generic pathway for plant response to stress. The extracellular stress signal is first perceived by the membrane receptors and then activate large and complex signaling cascade intracellularly including the generation of secondary signal molecules. The stress signal can first activates phospholipase C (PLC), which hydrolyses PIP2 to generate IP3 and DAG resulting in an increase in the level of Ca2+ ions in the cytosol, which is sensed by calcium sensor. The signal cascade results into the expression of multiple stress responsive genes, the products of which can provide the stress tolerance directly or indirectly. Overall, the stress response could be coordination action of many genes, which may cross-talk with each others.
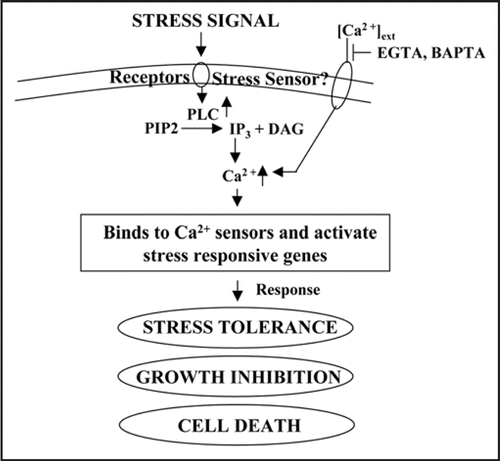
Figure 2 The units of the calcium signaling network. A major protein that regulates a number of calcium mediated signals is calmodulin, which has four calcium binding sites (A). On receiving stimulii, calcium mobilizing signals act on various ON mechanisms that lead to an increase in the intracellular Ca++. The increase in calcium is sensed by various proteins like calmodulin or directly by calcium dependent protein kinases (CDPKs). The CDPKs have a catalytic domain and calcium binding domain like calmodulin (B). There are other calcium binding proteins also which can sense calcium concentration. All these proteins can trigger downstream processes which can elicit specific response (C). The response is terminated by OFF mechanisms that restore Ca++ to the resting level.
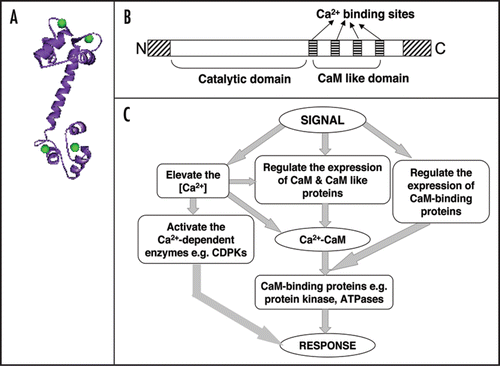
Figure 3 NO as a signaling molecule. The NO is synthesized from arginine (A). In plants the NO is synthesized by an enzyme that has homology with the p-protein of alanine decarboxylase in response to pathogen. It can also be synthesized by the activity of nitrate reductase as was shown in ABA mediated stomata closure response (B).
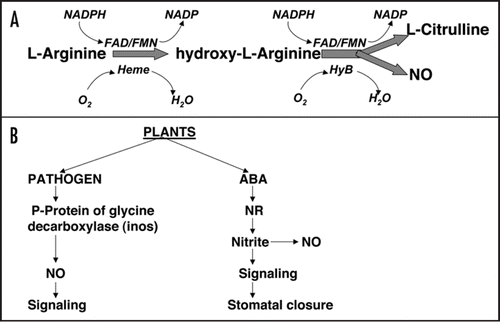
Acknowledgements
This work was partially supported by the grants from the Department of Biotechnology and Department of Science and Technology, Government of India. We apologize if some references could not been cited due to space constraint.
References
- Bray EA, Bailey Serres J, Weretilnyk E. Gruissem W, Buchannan B, Jones R. Responses to abiotic stresses. Biochemistry and Molecular Biology of Plants 2000; Rockville, MD American Society of Plant Biologists 158
- Trewavas AJ, Malho R. Signal perception and Transduction: The origin of the phenotype. Plant Cell 1997; 9:1181 - 1195
- McCarty DR, Chory J. Conservation and innovation in plant signaling pathway. Cell 2000; 103:201 - 209
- Gilroy S, Trewavas A. Signal processing and Transduction in plant cell: the end of the beginning. Nature Rev Mol Cell Biol 2001; 2:307 - 314
- Sopory SK, Oelmueller R, Maheshwari SC. Signal Transduction in plants: Current Advances 2001; NY Kluwer Academic/Plenum Publisher
- Mahajan S, Tuteja N. Cold, salinity and drought stresses: An overview. Arch Biochem Biophys 2005; 444:139 - 158
- Tuteja N. Mechanisms of high salinity tolerance in plants. Methods Enzymol 2007; 428:419 - 438
- Tuteja N, Mahajan S. Calcium signaling network in plants: an overview. Plant Signal Behav 2007; 2:79 - 85
- Tuteja N. Abscisic acid and abiotic stress signalling. Plant Signal Behav 2007; 2:135 - 138
- Pardo JM, Reddy MP, Yang S, Maggio A, Huh GH, Matsumoto T, Coca MA, D'urzo MP, Koiwa H, Yun DJ, Watad A, Bressan RA, Hasegawa PM. Stress signaling through Ca2+/calmodulin-dependent protein phosphatase calcineurine mediates salt adaptation in plant. Proc Natl Acad Sci USA 1998; 95:9681 - 9686
- Chory J, Wu D. Weaving the complex web of signal transduction. Plant Physiol 2001; 125:77 - 80
- Reddy ASN. Calcium: silver bullet in signaling. Plant Sci 2001; 160:612 - 619
- Mahajan S, Sopoy SK, Tuteja N. CBL-CIPK paradigm: role in calcium and stress signaling in plants. Proc Indian Natl Sci Acad 2006; 72:63 - 78
- Bush DS. Calcium regulation in plant cells and its role in signaling. Annu Rev Plant Physiol Plant Mol Biol 1995; 46:95 - 122
- Takano M, Takahashi H, Suge H. Calcium requirement for the induction of hydrogen of hypotropism abd enhancenment of calcium induced curvature by water stress in primary roots of pea Pisum sativum L.. Plant Cell Physiol 1997; 38:385 - 391
- Gong M, Van del Luit AH, Knight MR, Trewavas AJ. Heat shock induced change in intracellular calcium level in tobacco seedlings in relation to thermo tolerance. Plant Physiol 1998; 116:429 - 437
- Kinight H, Trewavas AJ, Knight MR. Calcium signaling in Arabidopsis thaliana responding to drought and salinity. Plant J 1997; 12:1067 - 1078
- Kiegle E, Moore CA, Haseloff J, Tester MA, Knight MR. Cell-type-specific calcium responses to drought, salt and cold in the Arabidopsis root. Plant J 2000; 23:267 - 278
- Pandey S, Tiwari SB, Upadhyaya KC, Sopory SK. Calcium signaling: linking environmental signals to cellular functions. Crit Rev Plant Sci 2000; 19:291 - 318
- Pandey S, Tiwari SB, Tyagi W, Reddy MK, Upadhyaya KC, Sopory SK. A Ca2+/CaM-dependent kinase from pea is stress regulated and in vitro phosphorylates a protein that binds to AtCaM5 promoter. Eur J Biochem 2002; 269:3193 - 3204
- Verdus MC, Sceller LC, Norris V, Thellier M, Ripoll C. Pharmacological evidence for Calcium Involvement in the long-term processing of abiotic stimuli in plant. Plant Signal Behav 2007; 2:212 - 220
- Rudd JJ, Franklin Tong VE. Unravelling response-specificity in Ca2+ signalling pathways in plant cells. New Phytol 2001; 151:7 - 33
- Klimecka M, Muszynska G. Structure and functions of plant calcium-dependent protein kinases. Acta Biochimica Polonica 2007; 54:219 - 233
- Luan S, Kudla J, Rodríguez Concepción M, Yalovsky S, Gruissem W. Calmodulins and calcineurin B-like proteins: calcium sensors for specific signal response coupling in plants. Plant Cell 2002; 14:389 - 400
- Vanderbeld B, Snedden WA. Developmental and stimulus-induced expression patterns of Arabidopsis calmodulin-like genes CML37, CML38 and CML39. Plant Mol Biol 2007; 64:683 - 697
- Wang X. Plant phospholipases. Annu Rev Plant Physiol Plant Mol Biol 2001; 52:211 - 231
- Ritchie SM, Swanson SJ, Gilroy S. From common signalling components to cell specific responses: insights from the cereal aleurone. Physiologia Plantarum 2002; 115:342 - 351
- Górecka KM, Trebacz K, Górecki R, Pikula S. Participation of annexin At1 in plant response to abiotic stress. Postepy Biochem (Polish) 2007; 53:154 - 158
- Gorecka KM, Thouverey C, Buchet R, Pikula S. Potential role of annexin AnnAt1 from Arabidopsis thaliana in pH-mediated cellular response to environmental stimuli. Plant Cell Physiol 2007; 48:792 - 803
- Ehtesham NZ, Phan TN, Gaikwad A, Sopory SK, Tuteja N. Calnexin from Pisum sativum: cloning of the cDNA and characterization of the encoded protein. DNA Cell Biol 1999; 18:853 - 862
- Sarwat M, Tuteja N. Calnexin: a versatile calcium binding integral membrane-bound chaperone of endoplasmic reticulum. Calcium Binding Proteins 2007; 2:36 - 50
- Kaplan B, Davydov O, Knight H, Galon Y, Knight MR, Fluhr R, Fromm H. Rapid Transcriptome Changes Induced by Cytosolic Ca2+ Transients Reveal ABRE-Related Sequences as Ca2+-Responsive cis Elements in Arabidopsis. Plant Cell 2006; 18:2733 - 2748
- Bouché N, Scharlat A, Snedden W, Bouchez D, Fromm H. A novel family of calmodulin-binding transcription activators in multicellular organisms. J Biol Chem 2002; 277:21851 - 21861
- Yang T, Poovaiah BW. A calmodulin-binding/CGCG box DNA-binding protein family involved in multiple signaling pathways in plants. J Biol Chem 2002; 277:45049 - 45058
- Choi MS, Kim MC, Yoo JH, Moon BC, Koo SC, Park BO, Lee JH, Koo YD, Han HJ, Lee SY, Chung WS, Lim CO, Cho MJ. Isolation of a calmodulin-binding transcription factor from rice (Oryza sativa L.). J Biol Chem 2005; 280:40820 - 40831
- Finkler A, Ashery Padan R, Fromm H. CAMTAs: calmodulin-binding transcription activators from plants to human. FEBS Lett 2007; 581:3893 - 3898
- Mahajan S, Pandey GK, Tuteja N. Calcium and salt stress signaling in plants: shedding light on SOS pathway. Arch Biochem Biophys 2008; 471:146 - 158
- Liu J, Zhu JK. Proline accumulation and salt-stress-induced gene expression in a salt-hypersensitive mutant of Arabidopsis. Plant Physiol 1997; 114:591 - 596
- Zhu JK. Genetic analysis of plant salt tolerance using Arabidopsis. Plant Physiol 2000; 124:941 - 948
- Zhu JK, Liu J, Xiong L. Genetic analysis of salt tolerance in Arabidopsis. Evidence for a critical role of potassium nutrition. Plant Cell 1998; 10:1181 - 1191
- Kohler C, Merkle T, Neuhaus G. Characterization of a novel gene family of putative cyclic nucleotide and calmodulin-regulated ion channels in Arabidopsis thaliana. Plant J 1999; 18:97 - 104
- Maathuis FJ, Sanders D. Sodium uptake in Arabidopsis roots is regulated by cyclic nucleotides. Plant Physiol 2001; 127:1617 - 1625
- Maathuis FJ. cGMP modulates gene transcription and cation transport in Arabidopsis roots. Plant J 2006; 45:700 - 711
- Pical C, Westergren T, Dove SK, Larsson C, Sommarin M. Salinity and hyperosmotic stress induce rapid increases in phosphatidylinositol 4,5-bisphosphate, diacylglycerol pyrophosphate, and phosphatidylcholine in Arabidopsis thaliana cells. J Biol Chem 1999; 274:38232 - 38240
- Prescott SM. A thematic series on kinases and phosphatases that regulate lipid signaling. J Biol Chem 1999; 274:8345 - 8346
- Drobak BK, Watkins PAC. Inositol(1,4,5)trisphosphate production in plant cells: an early response to salinity and hyperosmotic stress. FEBS Letters 2000; 481:240 - 244
- Chapman KD. Phospholipase activity during plant growth and development and in response to environmental stress. Trends Plant Sci 1998; 3:419 - 426
- Tuteja N, Sopory SK. Plant signaling in stress: G-protein coupled receptors, heterotrimeric G-proteins and signal coupling via phospholipases. Plant Signal Behav 2008; 3:79 - 86
- Vanhaesebroeck B, Leevers SJ, Panayotou G, Waterfield MD. Phosphoinositide 3-kinases: a conserved family of signal transducers. Trends Biochem Sci 1997; 22:267 - 272
- Welters P, Takegawa K, Emr SD, Chrispeels MJ. AtVPS34, a phosphatidylinositol 3-kinase of Arabidopsis thaliana, is an essential protein with homology to a calcium-dependent lipid binding domain. Proc Natl Acad Sci 1994; 91:11398 - 11402
- Hong Z, Verma DP. A phosphatidylinositol 3-kinase is induced during soybean nodule organogenesis and is associated with membrane proliferation. Proc Natl Acad Sci 1994; 91:9617 - 9621
- Bunney TD, Watkins PAC, Beven AF, Shaw PJ, Hernandez LE, Lomonossoff GP, Shanks M, Peart J, Drøbak BK. Association of phosphatidylinositol 3-kinase with nuclear transcription sites in higher plants. Plant Cell 2000; 12:1679 - 1688
- Park KY, Jung JY, Park J, Hwang JU, Kim YW, Hwang I, Lee Y. A role for phosphatidylinositol 3-phosphate in abscisic acid-induced reactive oxygen species generation in guard cells. Plant Physiol 2003; 132:92 - 98
- Joo JH, Yoo HJ, Hwang I, Lee JS, Nam KH, Bae YS. Auxin-induced reactive oxygen species production requires the activation of phosphatidylinositol 3-kinase. FEBS Lett 2005; 579:1243 - 1248
- Leshem Y, Seri L, Levine A. Induction of phosphatidylinositol 3-kinase-mediated endocytosis by salt stress leads to intracellular production of reactive oxygen species and salt tolerance. Plant J 2007; 51:185 - 197
- Testernik C, Munnik T. Phosphatidic acid: a multifunctional stress signaling lipid in plants. Trend Pl Sci 2005; 10:368 - 375
- Wang X, Devaiah SP, Zhang W, Welti R. Signaling functions of phosphatidic acid. Prog lipid Res 2006; 45:250 - 278
- Meijer HJ, Munnik T. Phospholipid-based signaling in plants. Annu Rev Plant Biol 2003; 54:265 - 306
- Testerink C, Larsen PB, van der Does D, van Himbergen JA, Munnik T. Phosphatidic acid binds to and inhibits the activity of Arabidopsis CTR1. J Exp Bot 2007; 58:3905 - 3914
- van Schooten B, Testernik C, Munnik T. Signalling diacylglycerol pyrophosphate, a new phosphatidic acid metabolite. Biochem Biophys Acta 2006; 1761:151 - 159
- Konig S, Mosblech A, Helimann I. Stress-inducible and constitutive phosphoinositide pools have distinctive fatty acid patterns in Arabidopsis thaliana. FASEB J 2007; 21:1958 - 1967
- Takahashi S, Katagiri T, Hirayama T, Yamaguchi Shinozaki K, Shinozaki K. Hyperosmotic stress induces a rapid and transient increase in inositol 1,4,5-trisphosphate independent of abscisic acid in Arabidopsis cell culture. Plant Cell Physiol 2001; 42:214 - 222
- Chandok MR, Sopory SK. ZmcPKC70, A protein kinase C-type enzyme from maize: biochemical characterization, regulation by phorbol myristate acetate and its possible involvement in nitrate reductase gene expression. J Biol Chem 1998; 273:19235 - 19242
- Parre E, Ghars MA, Leprince AS, Thiery L, Lefebvre D, Bordenave M, Richard L, Mazars C, Abdelly C, Savouré A. Calcium signaling via phospholipase C is essential for proline accumulation upon ionic but not nonionic hyperosmotic stresses in Arabidopsis. Plant Physiol 2007; 144:503 - 512
- Tang RH, Han S, Zheng H, Cook CW, Choi CS, Woerner TE, Jackson RB, Dei ZM. Coupling diurnal cytosolic Ca2+ oscillations to the CAS-IP3 pathway in Arabidopsis. Science 2007; 315:1423 - 1426
- Tuteja N, Chandra M, Tuteja R, Misra MK. Nitric oxide as a unique bioactive signaling messenger in physiology and pathophysiology. J Biomed Biotech 2004; 2004:227 - 237
- Romero Puertas MC, Delledonne M. Nitric oxide signaling in plant-pathogen interactions. IUBMB Life 2003; 55:579 - 583
- Correa Aragunde N, Graziano M, Lamattina L. Nitric oxide plays a central role in determining lateral root development in tomato. Planta 2004; 218:900 - 905
- Huang X, Stettmaier K, Michel C, Hutzler P, Mueller MJ, Durner J. Nitric oxide is induced by wounding and influences jasmonic acid signaling in Arabidopsis thaliana. Planta 2004; 218:938 - 946
- Grün S, Lindermayr C, Sell S, Durner J. Nitric oxide and gene regulation in plants. J Exp Bot 2006; 57:507 - 516
- Ali R, Ma W, Lemtiri Chlieh F, Tsaltas D, Leng Q, von Bodman S, Berkowitz GA. Death don't have no mercy and neither does calcium: Arabidopsis CYCLIC NUCLEOTIDE GATED CHANNEL2 and innate immunity. Plant Cell 2007; 19:1081 - 1095
- Laxalt AM, Raho N, Have AT, Lamattina L. Nitric oxide is critical for inducing phosphatidic acid accumulation in xylanase-elicited tomato cells. J Biol Chem 2007; 282:21160 - 21168
- Graziano M, Lamattina L. Nitric oxide accumulation is required for molecular and physiological responses to iron deficiency in tomato roots. Plant J 2007; 52:949 - 960
- Besson Bard A, Courtois C, Gauthier A, Dahan J, Dobrowolska G, Jeandroz S, Pugin A, Wendenhenne D. Nitric oxide in plants: production and cross talk with Ca2+ signaling. Mol Plant 2008; 1:218 - 228
- Zhang F, Wang Y, Wang D. Role of Nitric Oxide and Hydrogen Peroxide During the Salt Resistance Response. Plant Signal Behav 2007; 2:473 - 474
- Distéfano AM, García Mata C, Lamattina L, Laxalt AM. Nitric oxide-induced phosphatidic acid accumulation: a role for phospholipases C and D in stomatal closure. Plant Cell Environ 2008; 31:187 - 194
- Baier M, Hemmann G, Holman R, Corke F, Card R, Smith C, Rook F, Bevan MW. Characterization of mutants in Arabidopsis showing increased sugar-specific gene expression, growth, and developmental responses. Plant Physiol 2004; 134:81 - 91
- Rolland F, Baena Gonzalez E, Sheen J. Sugar sensing and signaling in plants: conserved and novel mechanisms. Annu Rev Plant Biol 2006; 57:675 - 709
- Cho YH, Yoo SD, Sheen J. Regulatory functions of nuclear hexokinase1 complex in glucose signaling. Cell 2006; 127:579 - 589
- Chen JG. Sweet sensor, surprising partners. Sci STKE 2007; 2007:7
- Li Y, Smith C, Corke F, Zheng L, Merali Z, Ryden P, Derbyshire P, Waldron K, Bevan MW. Signaling from an Altered Cell Wall to the Nucleus Mediates Sugar-Responsive Growth and Development in Arabidopsis thaliana. Plant Cell 2007; 19:2500 - 2515
- Qiu QS, Hardin SC, Mace J, Brutnell TP, Huber SC. Light and metabolic signals control the selective degradation of sucrose synthase in maize leaves during deetiolation. Plant Physiol 2007; 144:468 - 478
- Kempa S, Rozhon W, Samaj J, Erban A, Baluska F, Becker T, Haselmayer J, Schleiff E, Kopka J, Hirt H, Jonak C. A plastid-localized glycogen synthase kinase 3 modulates stress tolerance and carbohydrate metabolism. Plant J 2007; 49:1076 - 1090
- Baena Gonzalez E, Rolland F, Thevelein JM, Sheen J. A central integrator of transcription network in plant stress and energy signaling. Nature 2007; 448:938 - 942
- Tran LS, Urao T, Qin F, Maruyama K, Kakimoto T, Shinozaki K, Yamaguchi-Shinozaki K. Functional analysis of AHK1/ATHK1 and cytokinin receptor histidine kinases in response to abscisic acid, drought, and salt stress in Arabidopsis. Proc Natl Acad Sci USA 2007; 104:20623 - 20628
- Park JE, Seo PJ, Lee AK, Jung JH, Kim YS, Park CM. An Arabidopsis GH3 gene, encoding an auxin-conjugating enzyme, mediates phytochrome B-regulated light signals in hypocotyl growth. Plant Cell Physiol 2007; 48:1236 - 1241
- Park JE, Park JY, Kim YS, Staswick PE, Jeon J, Yun J, Kim SY, Kim J, Lee YH, Park CM. GH3-mediated auxin homeostasis links growth regulation with stress adaptation response in Arabidopsis. J Biol Chem 2007; 282:10036 - 10046
- Jackson MB. Ethylene-promoted elongation: an adaptation to submergence stress. Ann Bot (Lond) 2008; 101:229 - 248
- Krishna P. Brassinosteroid-mediated stress response. J Plant Growth Regul 2003; 22:289 - 297
- Kagale S, Divi UK, Krochko JE, Keller WA, Krishna P. Brassinosteroid confers tolerance in Arabidopsis thaliana and Brassica napus to a range of abiotic stresses. Planta 2007; 225:353 - 364
- Baldwin IT, Kessler A, Halitschke R. Volatile signaling in plant-plant-herbivore interactions: what is real?. Curr Opin Plant Biol 2002; 5:351 - 354
- Bruce TJ, Wadhams LJ, Woodcock CM. Insect host location: a volatile situation. Trends Plant Sci 2005; 10:269 - 274
- Loreto F, Barta C, Brilli F, Nogues I. On the induction of volatile organic compound emissions by plants as consequence of wounding or fluctuations of light and temperature. Plant Cell Environ 2006; 29:1820 - 1828
- Tripathi SK, Tuteja N. Integrated signaling in flower senescence: an overview. Plant Signal Behav 2007; 2:437 - 445
- Swamy PM, Smith B. Role of abscisic acid in plant stress tolerance. Curr Sci 1999; 76:1220 - 1227
- Koornneef M, Leon Kloosterziel KM, Schwartz SH, Zeevaart JAD. The genetic and molecular dissection of abscisic acid biosynthesis and signal transduction in Arabidopsis. Plant Physiol Biochem 1998; 36:83 - 89
- Xiong L, Ishitini M, Lee H, Zhu JK. The Arabidopsis LOS5/ABA3 locus encodes a molybdenum cofactor sulfurase and modulates cold stress and osmotic stress responsive gene expression. Plant Cell 2001; 13:2063 - 2083
- Yamaguchi Shinozaki K, Shinozaki K. Characterization of the expression of a desiccation-responsive rd29 gene of Arabidopsis thaliana and analysis of its promoter in transgenic plants. Mol Gen Genet 1993; 236:331 - 340
- Park CM. Auxin homeostasis in plant stress adaptation response. Plant Signal Behav 2007; 2:306 - 307
- Sanan Mishra N, Phan XH, Sopory SK, Tuteja N. Pea DNA helicase 45 overexpression in tobacco confers high salinity tolerance without affecting yield. Proc Natl Acad Sci USA 2005; 102:509 - 514
- Mahajan S, Sopoy SK, Tuteja N. Cloning and characterization of CBL-CIPK signaling components from a legume (Pisum sativum). FEBS J 2006; 273:907 - 925
- Xiong L, Wang RG, Mao G, Koczan JM. Identification of drought tolerance determinants by genetic analysis of root response to drought stress and abscisic Acid. Plant Physiol 2006; 142:1065 - 1074
- Cao X, Costa LM, Biderre Petit C, Kbhaya B, Dey N, Perez P, McCarty DR, Gutierrez Marcos JF, Becraft PW. Abscisic acid and stress signals induce Viviparous1 expression in seed and vegetative tissues of maize. Plant Physiol 2007; 143:720 - 731
- Razem FA, El Kereamy A, Abrams SR, Hill RD. The RNA-binding protein FCA is an abscisic acid receptor. Nature 2006; 439:290 - 294
- Zhang JF, Yuan LJ, Shao Y, Du W, Yan DW, Lu YT. The disturbance of small RNA pathways enhanced abscisic acid response and multiple stress responses in Arabidopsis. Plant Cell Environ 2008; 31:562 - 574
- Wasternack C. Jasmonates: An update on biosynthesis, signal transduction and action in plant stress response, growth and development. Ann Bot (Lon) 2007; 100:681 - 697
- Seo S, Katou S, Seto H, Gomi K, Ohashi Y. The mitogen-activated protein kinases WIPK and SIPK regulate the levels of jasmonic and salicylic acids in wounded tobacco plants. Plant J 2007; 49:899 - 909
- Truman W, Bennett MH, Kubigsteltig I, Turnbull C, Grant M. Arabidopsis systemic immunity uses conserved defense signaling pathways and is mediated by jasmonates. Proc Natl Acad Sci USA 2007; 104:1075 - 1080
- Walia H, Wilson C, Condamine P, Liu X, Ismail AM, Close TJ. Large-scale expression profiling and physiological characterization of jasmonic acid-mediated adaptation of barley to salinity stress. Plant Cell Environ 2007; 30:410 - 421
- Dat JF, Lopez Delgado H, Foyer CH, Scott IM. Parallel changes in H2O2 and catalase during thermotolerance induced by salicylic acid or heat acclimation in mustard seedlings. Plant Physiol 1998; 116:1351 - 1357
- Borsani O, Valpuesta V, Botella MA. Evidence for a role of salicylic acid in the oxidative damage generated by NaCl and osmotic stress in Arabidopsis seedlings. Plant Physiol 2001; 126:1024 - 1030
- Tyagi W, Rajagopal D, Singla Pareek SL, Reddy MK, Sopory SK. Cloning and regulation of a stress-regulated Pennisetum glaucum vacuolar ATPase c gene and characterization of its promoter that is expressed in shoot hairs and floral organs. Plane Cell Physiol 2005; 46:1411 - 1422
- Ndamukong I, Abdallat AA, Thurow C, Fode B, Zander M, Weigel R, Gatz C. SA-inducible Arabidopsis glutaredoxin interacts with TGA factors and suppresses JA-responsive PDF1.2 transcription. Plant J 2007; 50:128 - 139
- Koo YJ, Kim MA, Kim EH, Song JT, Jung C, Moon JK, Kim JH, Seo HS, Song SI, Kim JK, Lee JS, Cheong JJ, Choi YD. Overexpression of salicylic acid carboxyl methyltransferase reduces salicylic acid-mediated pathogen resistance in Arabidopsis thaliana. Plant Mol Biol 2007; 64:1 - 15
- Wang D, Pajerowska Mukhtar K, Culler AH, Dong X. Salicylic Acid Inhibits Pathogen Growth in Plants through Repression of the Auxin Signaling Pathway. Curr Biol 2007; 17:1784 - 1790
- Jiménez Bremont JF, Ruiz OA, Rodríguez Kessler M. Modulation of spermidine and spermine levels in maize seedlings subjected to long-term salt stress. Plant Physiol Biochem 2007; 45:812 - 821
- Kusano T, Yamaguchi K, Berberich T, Takahashi Y. Advances in polyamine research in 2007. J Plant Res 2007; 120:345 - 350
- Groppa MD, Benavides MP. Polyamines and abiotic stress: recent advances. Amino Acids 2008; 34:35 - 45
- Yamaguchi K, Takahashi Y, Berberich T, Imai A, Miyazaki A, Takahashi T, Michael A, Kusano T. The polyamine spermine protects against high salt stress in Arabidopsis thaliana. FEBS Letters 2006; 580:6783 - 6788
- Yamaguchi K, Takahashi Y, Berberich T, Imai A, Takahashi T, Michael AJ, Kusano T. A protective role for the polyamine spermine against drought stress in Arabidopsis. Biochem Biophys Res Commun 2007; 352:486 - 490
- Zhao F, Song CP, He J, Zhu H. Polyamine improve K+/Na+ homeostasis in barley seedlings by regulating root ion channel activities. Plant Physiol 2007; 145:1061 - 1072
- Bae H, Kim SH, Kim MS, Sicher RC, Lary D, Strem MD, Natarajan S, Bailey BA. The drought response of Theobroma cacao (cacao) and the regulation of genes involved in polyamine biosynthesis by drought and other stresses. Plant Physiol Biochem 2008; 46:174 - 188
- Yamasaki H, Cohen MF. NO signal at the cross roads: polyamine induced nitric oxide synthesis in plants?. Trends Plant Sci 2006; 11:522 - 524
- Sano T, Higaki T, Handa K, Kadota Y, Kuchitsu K, Hasezawa S, Hoffmann A, Endter J, Zimmermann U, Hedrich R, Roitsch T. Calcium ions are involved in the delay of plant cell cycle progression by abiotic stresses. FEBS Lett 2006; 580:597 - 602
- Paul M. Trehalose 6-phosphate. Current Opin Plant Biol 2007; 10:303 - 309
- Shao HB, Chu LY, Lu ZH, Kang CM. Primary antioxidant free radical scavenging and redox signaling pathways in higher plant cells. Int J Biol Sci 2007; 4:8 - 12
- Neill S, Bright J, Desikan R, Hancock J, Harrison J, Wilson I. Nitric oxide evolution and perception. J Exp Bot 2008; 59:25 - 35