Abstract
MicroRNAs (miRNAs) are ubiquitous regulators of gene expression that contribute to almost any cellular process. Methods for managing of miRNA activity are attracting increasing attention in relation to diverse experimental and therapeutic applications. DNA-encoded miRNA inhibitors expressed from plasmid or virus-based vectors provide persistent miRNA suppression and options of tissue-directed micromanaging. In this report, we explore the potential of exploiting short, hairpin-shaped RNAs for simultaneous suppression of two or more miRNAs. Based on the “Tough Decoy” (TuD) design, we create dual-targeting hairpins carrying two miRNA recognition sites and demonstrate potent co-suppression of different pairs of unrelated miRNAs by a single DNA-encoded inhibitor RNA. In addition, enhanced miRNA suppression is achieved by expression of RNA polymerase II-transcribed inhibitors carrying clustered TuD hairpins with up to a total of eight miRNA recognition sites. Notably, by expressing clustered TuD inhibitors harboring a single recognition site for each of a total of six miRNAs, we document robust parallel suppression of multiple miRNAs by inhibitor RNA molecules encoded by a single expression cassette. These findings unveil a new potential of TuD-based miRNA inhibitors and pave the way for standardizing synchronized suppression of families or clusters of miRNAs.
Introduction
As ubiquitous regulators of gene expression, microRNAs (miRNAs) influence the regulation of almost any cellular process, including cell proliferation, differentiation, metabolism and apoptosis. Not surprisingly, disturbed miRNA expression is associated with development of disease, including a variety of cancers,Citation1-Citation3 and potent methods for managing miRNAs are increasingly important in basic studies of disease development with potential applications also in genetically based treatment of disease. MiRNAs are short, non-coding RNAs [from 20–24 nucleotides (nt) long] that regulate gene expression post-transcriptionally by binding to mRNAs, most often through imperfect basepairing. This interaction, frequently involving the 3′ untranslated region (UTR) of the mRNA, triggers mRNA cleavage or translational repression facilitated by components of the RNA-induced silencing complex (RISC).
Inhibitors of miRNA function can be roughly divided in two major classes; synthetic oligonucleotides and DNA-encoded short RNAs. Both classes of inhibitors exploit the complementarity to fully processed miRNAs to specifically target and out-titrate miRNAs of interest. So far, chemically modified antisense oligonucleotides have attracted the most attention due to their capacity to efficiently suppress miRNAs in vivo.Citation4 Intravenous administration of such “antagomirs” induces a transient response, and repeated administration is therefore required for persistent miRNA suppression. In addition, systemic delivery of synthetic oligonucleotides may pose an inherent risk of regulating miRNAs in tissues that are not relevant for a given treatment and potentially cause toxicity due to unintended off-target effects. DNA-encoded miRNA inhibitors, RNA molecules expressed from plasmid or viral vector DNA, represent an intriguing alternative that may offer increased tissue-specificity and persistency of targeted miRNA intervention. Hence, delivery of such inhibitors will benefit from advanced gene transfer technologies and strategies of tissue-directed gene delivery that have been developed for gene therapy application.Citation5
The simplest type of DNA-encoded miRNA inhibitor is a short 20–24 nt RNA antagomiR with full complementarity to the processed miRNA. Although we and others have demonstrated targeted miRNA suppression by this type of inhibitor,Citation6,Citation7 several optimized inhibitor designs possess enhanced potency owing to high structural stability, improved miRNA accessibility and, in some cases, an increased number of miRNA recognition sequences per RNA molecule. Prominent inhibitors include “Bulged sponges” containing tandemly arranged miRNA-binding sites,Citation8 and hairpin-shaped Tough Decoys (TuDs) with a large internal loop containing two miRNA-binding sites.Citation9 Recently, we performed a side-by-side comparison of seven different DNA-encoded miRNA inhibitors and found that Bulged sponges and TuDs were the most potent miRNA inhibitors expressed from both transfected plasmid DNA and transduced lentiviral vectors.Citation10 The structure of DNA-encoded TuDs has been mimicked in synthetic TuD molecules that are known to inhibit miRNAs efficiently.Citation11 Both Bulged sponges and TuDs can be expressed as short RNA transcripts from an RNA polymerase III promoter or may alternatively be fused to a protein-encoding RNA and expressed from an RNA polymerase II promoter.Citation10,Citation12
In this study, we refine the design of DNA-encoded TuD miRNA inhibitors. We show increased potency of multiplexed inhibitors containing up to four tandemly arranged TuD hairpins and demonstrate effective simultaneous suppression of two distinct miRNAs by a single, dual-targeting TuD inhibitor carrying two miRNA recognition sequences. By combining these two methodologies, we demonstrate synchronous suppression of up to six pre-determined miRNAs by expressing a single inhibitor RNA with three clustered TuD hairpins harboring a total of six miRNA recognition sequences.
Results
Experimental design for evaluation of miRNA suppression by DNA-encoded TuD RNA hairpins
TuD miRNA inhibitors, first described by Haraguchi and colleagues,Citation9 possess a hairpin-like structure harboring a large internal loop containing two identical miRNA-binding sites with sequences complementary to the miRNA of interest. The miRNA-binding sites are flanked on both sides by stem structures (). Moreover, both miRNA-binding sites are designed with mismatches (a 4-nt insert opposite to position 10 and 11 of the miRNA), which upon binding to the miRNA, create a bulge that prevents cleavage of the miRNA-binding site by Argonaute 2 in RISC. In this report, we undertook several efforts (illustrated schematically in ) to improve the potency and applicability of DNA-encoded TuD inhibitors. By mimicking the design of standard Bulged Sponge inhibitors, we first created clusters of TuD hairpins containing one, two, three or four tandemly arrayed TuD hairpins in the 3′-UTR of an eGFP reporter gene (). Second, we engineered vectors from which RNA polymerase III-transcribed TuD inhibitors targeting two miRNAs were expressed (), and third we combined dual-targeting TuD inhibitors in a cluster of three distinct TuD hairpins () fused to eGFP mRNA, allowing simultaneous suppression of six miRNAs by this RNA transcript. The potency by which TuD expression vectors suppressed the relevant miRNAs was evaluated by dual luciferase reporter assays in which perfect miRNA target sequences were fused to the Renilla luciferase (Rluc) gene in a reporter plasmid containing both the Renilla and Firefly luciferase (Fluc) expression cassettes. By co-transfection of HEK293 cells with individual TuD expression vectors and the relevant Rluc/Fluc reporter plasmid DNA, suppression of miRNA activity was measured as an increase of the normalized Rluc expression rate (Rluc/Fluc ratio) compared with controls. Due to low endogenous expression of some of the miRNAs in HEK293 cells, miRNA-encoding plasmids were included for these particular miRNAs.
Figure 1. Schematic representation of DNA-encoded TuD inhibitor designs. (A) The stem-loop shaped structure of the TuD is composed of four elements: an 18-bp long stem, two miRNA-binding sites, a 26-nt long stem-loop structure connecting the miRNA binding sites and four 3-nt long linkers joining the two miRNA-binding sites with the stem-loop and the stem, respectively. In each of the miRNA-binding sites, a 4-nt long bulge is introduced between nucleotide 10 and 11 from the 5′-end of the miRNA to avoid perfect base-pairing with miRNAs. In cases where the TuD is transcribed from an RNA Pol II promoter a 2- to 4-nt 3′-overhang is generated at the stem of the mature TuD. (B) RNA Pol II-expressed TuD clusters. (C) Dual-targeting TuDs expressed as short RNA transcripts from an H1 RNA Pol III promoter. (D) Multiple-targeting TuDs expressed from plasmid DNA as eGFP fusion transcripts from a PGK RNA Pol II promoter.
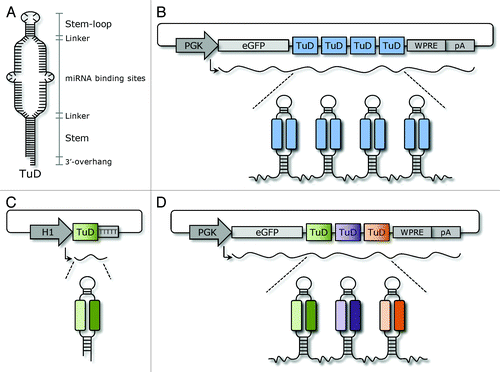
Inhibition of miRNAs by clustered TuD inhibitors
To compare the miRNA suppression potential of clustered TuD hairpins, we designed clusters containing one, two, three or four identical TuD hairpins targeting a single miRNA (). Among a total of five sets of vectors (targeting miR-143, -145, -195, -203 and -222, respectively), we measured in four cases significant miRNA suppression in the presence of a single TuD (eGFP-1xTuD) relative to expressed eGFP mRNA that did not contain a TuD hairpin (). Only in the case of miR-145, a single TuD (eGFP-1xTuD-145) was not sufficient to block the activity of the miRNA to an extent that triggered a significant increase in the level of Rluc ().
Figure 2. Suppression of miRNA activities by clustered TuD hairpins. (A) Schematic representation of TuD cluster transcripts. (B‒F) Levels of miRNA suppression as measured by increased Renilla luciferase expression from reporters expressing Rluc fused to the miRNA target sequence. Dual-Glo Luciferase assays were performed to monitor suppression of miR-195 (B), miR-222 (C), miR-143 (D), miR-145 (E) and miR-203 (F) activities by TuD clusters containing one, two, three and four tandemly arranged TuD hairpins. Data are depicted as mean + SEM.
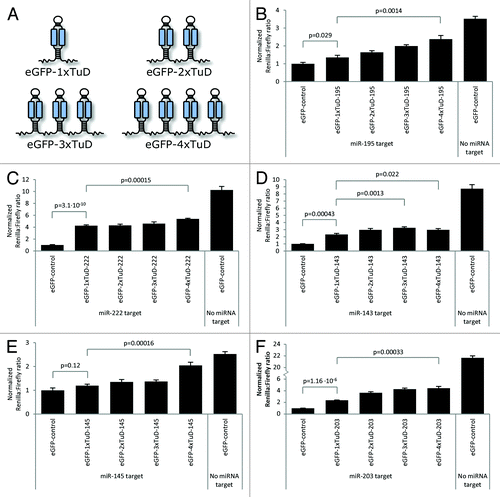
For all five miRNAs, improved miRNA suppression was observed for each TuD hairpin added to the cluster, as shown by increasing levels of Rluc expression with increasing number of TuD hairpins. Accordingly, all TuD clusters containing four tandemly arranged TuD hairpins (eGFP-4xTuD) resulted in levels of Rluc expression that were markedly increased relative to eGFP-1xTuD inhibitors (; p values ranging from 0.00015‒0.022). In case of miR143-directed TuD hairpins, we observed highest suppression by transcripts carrying three TuD hairpins (compare eGFP-1xTuD-143 and eGFP-3xTuD-143 in ; p = 0.0013). We reproducibly observed a lack of stability of plasmid DNA containing four miR143-directed TuD hairpins (resulting in deletion of one or more TuD hairpins, as determined by restriction analysis; data not shown), providing a possible explanation for the reduced activity of eGFP-4xTuD-143 relative to eGFP-3xTuD-143. Of all clustered TuD inhibitors that we have created, only this particular plasmid showed evidence of instability. In summary, all miRNAs were suppressed more efficiently by clustered TuD hairpins as compared with the control harboring only a single TuD hairpin.
Potent inhibition of two miRNAs by dual-targeting Tough decoys
Decoy miRNA inhibitors harboring two or more recognition sites for a specific miRNA have previously been shown to inhibit families of related miRNAs.Citation9,Citation11-Citation13 Here, we wanted to explore the potential of TuD hairpins to target and suppress two unrelated miRNAs. To this end, we designed dual-targeting TuD inhibitors carrying two heterologous miRNA recognition sites creating the large internal loop in the TuD stem. Vectors expressing such dual-targeting TuD hairpins recognizing miR-143 and -145 (TuD-143/145), miR-146a and -203 (TuD-203/146a) and miR-16 and -21 (TuD-16/21), respectively (schematically represented in middle panels of ), were tested for their ability to repress miRNA activity. As a positive control for suppression of each of the six miRNAs, we transfected plasmid DNA encoding a single mono-targeting TuD hairpin containing two identical miRNA-binding sites.
Figure 3. Efficient co-suppression of miRNAs by dual-targeting TuD inhibitors. (A) Co-suppression of miR-143 and miR-145 by dual-targeting TuD hairpins. (B) Co-suppression of miR-203 and miR-146a by dual-targeting TuD hairpins. (C) Co-suppression of miR-16 and miR-21 by dual-targeting TuD hairpins. Schematic representations of dual-targeting and standard TuD transcripts are provided in the middle panels. Data are depicted as mean + SEM.
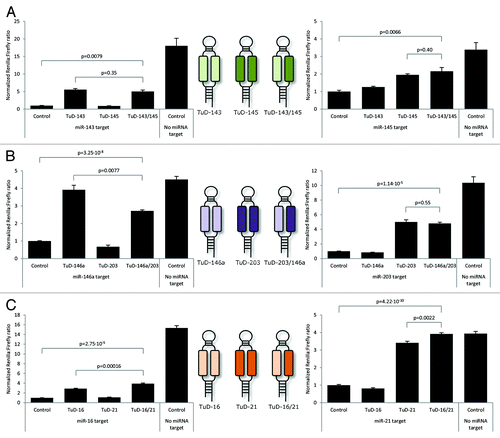
For each of the three dual-targeting TuD inhibitors, we observed robust suppression of the two targeted miRNAs (). For TuD-143/145, synchronous suppression of miR-143 and miR-145 was obtained to a level that was similar to the level obtained with the control TuDs targeting only a single miRNA (; p = 0.35 and p = 0.40 for targeting of miR-143 and miR-145, respectively). For TuD-146a/203, we observed a small reduction in suppression of miR-146a (, left panel; p = 0.0077), whereas miR-203 was equally suppressed by the dual-targeting TuD and the TuD-203 control (, right panel; p = 0.55). Notably, in case of the dual-targeting TuD directed against miR-16 and miR-21, we measured a significant increase in the expression level of the Rluc reporter gene relative to the level observed in the presence of TuD-16 and TuD-21, respectively (, left and right panels; p = 0.00016 and p = 0.0022). This finding demonstrated that the dual-targeting TuD-16/21 suppressed the activity of miR-16 and miR-21 more efficiently than TuD hairpins targeting each of the miRNAs.
The efficacy of TuD inhibitors is likely to rely on the accessibility of sequences in the large internal loop between the stem structures of the TuD hairpin (). It is of potential concern, therefore, if basepairing across the internal loop masks the miRNA recognition sequences and reduces the miRNA targeting capacity. To test if intrinsic activity of the TuD variants correlated with such potential basepairing, structures of mono- and dual-targeting TuD hairpins were predicted using CentroidFold,Citation14 which has previously been used for prediction of TuD structures.Citation11 As demonstrated by the predicted structures (provided in Fig. S1), potential basepairing between the two miRNA recognition sites could be detected for all TuD hairpins. However, we did not observe a clear correlation between the extent of basepairing and miRNA suppression capacity, and even TuD structures with an estimated high thermodynamic stability (like TuD-143, TuD-21 and TuD-16/21, ΔG values provided in Fig. S1) were able to act as efficient miRNA decoys.
In conclusion, we find that five out of six miRNAs were suppressed by dual-targeting TuD hairpins as efficiently as or even with higher efficiency than TuD inhibitors targeting a single miRNA. Hence, despite the fact that dual-targeting TuDs contain only a single recognition site for each miRNA, such inhibitors may easily be engineered to target two different miRNAs with high efficiency.
Simultaneous suppression of six different miRNAs by a multi-targeting TuD cluster
To address the applicability of TuD clusters for simultaneous suppression of the activity of two or more miRNAs, we first examined whether miRNA suppression was affected by the order of TuD hairpins in the cluster. For suppression of pairs of miRNAs (miR-143/-145 and miR-16/-21, respectively), we found that the order of the two TuDs each targeting a single of the two miRNAs did not influence miRNA suppression (data not shown). Also, the same level of suppression was obtained with inhibitors carrying two consecutive dual-targeting TuD hairpins targeting the same miRNAs (data not shown).
In an attempt to target several miRNAs from a single RNA transcript, we generated a vector expressing clustered dual-targeting TuD inhibitors targeting six distinct miRNAs in total. This miRNA suppression module consisted of three dual-targeting TuDs harboring miRNA-binding sites for miR-143 and -145, miR-146a and- 203 and miR-16 and -21, respectively (see for schematic illustration). The suppression potential of each of the TuD hairpins in the multi-targeting TuD cluster was evaluated in separate dual luciferase assays in which Rluc expression was regulated by each of the six target miRNAs (). For each of the miRNAs, the level of inhibition obtained with the multi-targeting TuD cluster was compared with the corresponding dual-targeting TuD hairpin. For all six miRNAs, the level of normalized Rluc expression in the presence of the clustered miRNA inhibitors was significantly higher than for eGFP negative control (; p values ranging from 5.85 × 10−8 to 7.1 × 10−4), indicating that all six miRNAs were efficiently suppressed by the eGFP mRNA transcript harboring the three TuD hairpins. For miR-145 and miR-21, the multi-targeting vector suppressed miRNA activity as efficiently as the corresponding dual-targeting vector (; p = 0.28 and p = 0.76, respectively), whereas dual-targeting TuD hairpins for the remaining four miRNAs blocked miRNA function were slightly more potent than the TuD cluster (; p -values ranging from 8.31 × 10−5 to 0.0039). In summary, our data demonstrate the feasibility of adapting DNA-encoded TuD inhibitors for potent and synchronous suppression of miRNAs. Hence, two miRNAs may be robustly suppressed by expression of a single dual-targeting TuD inhibitor, whereas multiple miRNAs may be targeted and suppressed by an RNA polymerase II-expressed mRNA transcript containing a cluster of dual-targeting TuD hairpins.
Figure 4. Synchronous suppression of six miRNAs by a multi-targeting clustered TuD inhibitor. (A) Schematic repre\sentation of the multi-targeting TuD cluster transcript containing three tandemly arranged dual-targeting TuDs targeting (1) miR-143 and miR-145, (2) miR-146a and miR-203 and (3) miR-16 and miR-21, respectively. (B‒G) Dual-Glo Luciferase assays evaluating the suppression of miR-143 (B), miR-145 (C), miR-146a (D), miR-203 (E), miR-16 (F) and miR-21 (G) by the multi-targeting TuD cluster. Data are depicted as mean + SEM.
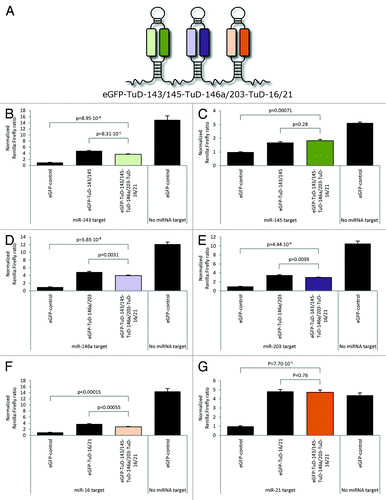
Discussion
Suppression of miRNA-directed gene regulation offer new opportunities in molecular experimentation and genetic therapies. As an example, synthetic miRNA inhibitors targeting miR-122 are currently investigated in the clinic for treatment of hepatitis C virus infections.Citation15,Citation16 Synthetic miRNA inhibitors can be distributed systemically and share the characteristics of classical medical drugs. Hence, treatment with such miRNA inhibitors may require repeated administration and may suffer from a low delivery rate in certain cell types. DNA-encoded miRNA inhibitors, in contrast, may benefit from higher persistency due to prolonged production of the inhibitor and may be expressed tissue-specifically from promoters with activities that are restricted to certain cell types.Citation17 Moreover, the use of vector-expressed miRNA inhibitors can be based on vector technologies developed and refined for gene therapy applications and may benefit from the recent success in this field. Hence, vector pseudotyping, for example, can support tissue-targeted activity of the inhibitors, reducing the inherent risk of off-target effects. Despite pros and cons for both synthetic and DNA-encoded inhibitors, the diverse experimental and therapeutic applications of miRNA suppression honor further optimization of structures and designs of both types of inhibitors.
We previously demonstrated efficacy of RNA polymerase II-transcribed TuD inhibitors fused to eGFP-encoding mRNA.Citation10 Notably, levels of miRNA suppression obtained with such TuD hairpins harboring two identical miRNA recognition sites were comparable to and for some miRNAs more efficient than RNA polymerase II-transcribed sponges harboring eight recognition sites.Citation10 These findings indicated that the functional properties, including structure and/or stability of the TuD hairpin embedded in a longer RNA transcript, were supportive for recruitment of cellular miRNAs. However, based on the current literature (reviewed in ref. Citation10) it remains uncertain whether targeting of miRNAs by TuD hairpins leads to miRNA degradation or miRNA sequestration, the latter which may suppress miRNA activity but also allow potential recycling of the miRNA. In the present study, we first showed that miRNA suppression could be further enhanced by including a cluster of more than one (up to four) tandemly arranged TuD hairpins in an eGFP fusion transcript. For all clustered TuD inhibitors tested in this study (targeting miR-143, -145, -195, -203 and -222, respectively), significantly higher levels of suppression were obtained with TuD clusters relative to a single TuD hairpin, unveiling benefits of TuD clusters mimicking the classical sponges. For one of the multiplexed TuD constructs, eGFP-4xTuD-143, we observed some plasmid instability, resulting in deletion of one or more TuD hairpins. This instability could be more pronounced in cases where TuD cassettes are delivered by retroviral transduction. Hence, rearrangements of direct repeat sequences during reverse transcription of vector RNACitation18 may lead to transduced cassettes with an increased or reduced number of TuD hairpins. Such events have been observed during transfer of conventional miRNA sponges with eight tandemly arrayed miRNA-binding sequences.Citation10
Synthetic or DNA-encoded TuDs and Bulges sponges targeting one particular miRNA have previously been shown to inhibit more members of a miRNA seed family,Citation9,Citation11-Citation13 but the ability of a single transcribed inhibitor to inhibit several unrelated miRNAs has remained unexplored. By expressing dual-targeting TuD hairpins harboring two recognition sites for two unrelated miRNAs, we observed synchronous suppression of these two miRNAs. However, it is not yet clear whether each dual-targeting TuD molecule is in fact able to bind two miRNAs or binds only one of the two targeted miRNAs. Surprisingly, even in the presence of only one miRNA recognition sequence within each expressed inhibitor, the suppression activity was comparable to or even higher than observed with TuD inhibitors targeting single miRNAs. This could potentially be ascribed to differences in the extent of basepairing between the two miRNA-binding sites in the standard TuDs compared with the dual-targeting TuDs. This notion corresponds to findings by Haraguchi et al., demonstrating that the accessibility of the TuD inhibitor to target miRNAs is impaired by potential basepairing between the two miRNA-binding sites in the TuD hairpin.Citation11 Introduction of single mutations or 4-nt insertions in the miRNA-binding sites was shown to be sufficient to increase the inhibitory function of TuDs with profound complementarity between the two miRNA-binding sites. In analogy, an inverse linear correlation between susceptibility of mRNA to degradation by small interfering RNA siRNA and stability of an RNA hairpin at the target site has previously demonstrated the importance of accessibility for specific RNA-RNA interactions leading to RNA interference.Citation19 Nevertheless, we were not able to detect a clear correlation between miRNA suppression activity and the predicted basepairing between the miRNA recognition sequences. An approach based on systematic destabilization of each specific TuD hairpin by mutagenesis is most likely required to describe such correlation in further detail. Most probably, the capacity of TuD hairpins to suppress miRNA activity is the combined result of creating minimal basepairing between the miRNA recognition sites in the TuD hairpin, allowing maximal miRNA accessibility, without disturbing basepairing and the specific interaction with miRNAs. Ways to achieve this balance between the optimal structure of the TuD and perfect basepairing to the miRNA will depend on the miRNA sequence and, therefore, may vary from miRNA to miRNA. In some cases, the TuD miRNA suppression capacity may potentially be further optimized by creating a hairpin structure with minimal basepairing within the internal loop, although such structure may contain only one recognition sequence with perfect miRNA complementarity.
The potency of dual-targeting TuD inhibitors as well as clustered TuDs allowed us to establish a multi-targeting DNA-encoded inhibitor with a cluster of three TuDs mediating simultaneous suppression of six unrelated miRNAs (miR-143, -145, -146a, -203, -16 and -21). This finding indicated that all miRNA-binding sites within the TuD cluster, at least in a population of eGFP mRNA transcripts, were accessible for miRNAs and, thus, were not sequestered by inappropriate folding of the clustered TuD hairpins. However, it cannot be ruled out that the miRNA-suppressing function of a particular TuD cluster containing multiple TuD hairpins may be impaired by inappropriate folding of the individual TuD hairpins depending on the sequences of the miRNA recognition sites.
In summary, in this study we refine the design of DNA-encoded TuD miRNA inhibitors. We show increased potency of clustered TuD inhibitors and demonstrate effective simultaneous suppression of two distinct miRNAs by a single, dual-targeting TuD inhibitor carrying two miRNA recognition sequences. By combining these two methodologies, we demonstrate synchronous suppression of up to six pre-determined miRNAs by RNA polymerase II-directed expression of a single inhibitor RNA with three clustered TuD hairpins holding a total of six miRNA recognition sequences. We believe that these new miRNA inhibitors are ideally suited for applications that require potent simultaneous suppression of two or more miRNAs.
Materials and Methods
Vector construction
TuD inhibitors that were expressed from an H1-promoter were inserted into the multiple cloning site of the expression vectors pCCL-PGK-eGFP-H1-MCS or pCCL-PGK-Puro-H1-MCS previously described in reference Citation20. Briefly, the TuD DNA sequences were synthesized and cloned into a pUC57 plasmid by GenScript. For cloning into AvrII/AscI-digested pCCL/PGK-eGFP-H1-MSC and pCCL/PGK-puro-H1-MSC, the TuD sequences were designed to include terminal NheI and AscI restriction sites. To create vectors expressing clustered TuD hairpins (1x, 2x, 3x and 4xTuD hairpins), the TuD sequences were PCR-amplified from pCCL/PGK-eGFP-H1-TuD or pCCL/PGK-puro-H1-TuD using primers flanking the TuD sequence. The 1xTuD PCR-fragments were inserted into the 3′-UTR of eGFP in pCCL/PGK-eGFPCitation21 using the KpnI restriction site, resulting in vectors designated pCCL/PGK-eGFP-1xTuD. The second TuD sequence was amplified by PCR and inserted at the 3′ border of the first TuD sequence in pCCL/PGK-eGFP-1xTuD using XbaI and MluI restriction sites, resulting in vectors designated pCCL/PGK-eGFP-2xTuD. Likewise, the third TuD PCR-fragments were inserted into pCCL/PGK-eGFP-2xTuD using XmaI and MluI restriction sites and the fourth by cloning into pCCL/PGK-eGFP-3xTuD using the MluI restriction site. Plasmid DNA encoding the multi-targeting TuD cluster (designated pCCL/PGK-eGFP-TuD-143/145-TuD-146a/203-TuD-16/21) was created like pCCL/PGK-eGFP-3xTuD vectors (see above).
psiCHECK vectors containing the Rluc gene fused to the perfect miRNA target site and the Fluc gene were made as previously described.Citation7 For each miRNA, complementary sense and antisense oligonucleotides were annealed by incubation at 100°C for 5 min followed by cooling at room temperature. The annealed oligonucleotides were designed for cloning into XhoI/NotI-digested psiCHECK-2 (Promega).
miRNA expression vectors for miR-143, -145, -146a and -203 were generated as previously described.Citation7 Briefly, the eGFP gene in the Sleeping Beauty DNA transposon vector pT2/CMV-eGFP(s)-SV40-neCitation22 was replaced by insertion of NotI-digested PCR amplicons containing the genomic region encompassing the pri-miRNA sequence. A PCR-fragment containing the miR-143/145 cluster was amplified from mouse genomic DNA, whereas miR-146a and miR-203 sequences were amplified from human genomic DNA using primers flanking the miRNA sequence. pT2/CMV-miR-143/145-SV40-hygro was generated from pT2/CMV-miR-143/145-SV40-neo by replacing the neomycin resistance gene with a hygromycin resistance gene. A part of the SV40 promoter and the hygromycin resistance gene were fused by an overlap extension PCR and inserted into BstBI/SfiI-digested pT2/CMV-miR-143/145-SV40-neo. The miR-221/222 gene cluster was PCR-amplified from mouse genomic DNA and inserted into pT2/CMV-miR-143/145-SV40-hygro by replacing the miR-143/145 cluster with the miR-221/222 cluster. Sequences of primers and TuDs are provided in and Tables S1‒3.
Cell culture
HEK293 cells were cultured at 37°C in 5% (v/v) C02 and maintained in Dulbecco’s modified Eagle’s medium (Cambrex) supplemented with 10% fetal calf serum, penicillin (100 U/mL), streptomycin (0.1 mg/mL) and L-glutamine (265 mg/L).
Dual-Glo luciferase assay
For analysis of miRNA suppression by Dual-Glo Luciferase Assays, HEK293 cells were seeded in white 96-well plates at a density of 3,000 cells/well 1 d prior to transfection. In studies of 1x, 2x, 3x and 4xTuD constructs targeting miR-143, -145 and -222, the cells were co-transfected with 12 ng TuD-encoding plasmid, 6 ng psiCHECK reporter and 3 ng of the miRNA expression vector. For suppression of miR-195, endogenously expressed miR-195 was targeted by TuD inhibitors and a miRNA expression vector was not included in the transfection mix. For suppression of miR-203, cells were transfected with 34 ng TuD-encoding plasmid, 6 ng psiCHECK reporter and 3 ng of the miRNA expression vector. Studies of dual-targeting TuD hairpins and the multi-targeting TuD vector (pCCL/PGK-eGFP-TuD-143/145-TuD-146a/203-TuD-16/21) were performed by transfection of 34 ng TuD-encoding plasmid, 6 ng psiCHECK reporter and 3 ng of the miRNA expression vector. For miR-16 and miR-21, endogenously expressed miRNAs were targeted and miRNA expression plasmid was not included in the transfection mix. All transfections were performed using FuGENE-6 according to the manufacturer’s protocol (Roche). Forty-eight hours post-transfection Rluc and Fluc activity was analyzed by the use of the Dual-Glo Luciferase Reporter Assay System (Promega) according to the manufacturer’s protocol. The luminescence reading was performed in a multisample platereading luminometer (Berthold). Rluc expression levels were normalized to the level of Fluc and presented relative to a negative control. All the experiments were performed at least in triplicates.
Abbrevitaions: miRNA | = | microRNA |
TuD | = | Tough Decoy |
nt | = | nucleotide |
UTR | = | untranslated region |
RISC | = | RNA-induced silencing complex |
Rluc | = | Renilla luciferase |
Fluc | = | Firefly luciferase |
siRNA | = | small interfering RNA |
Additional material
Download Zip (153.7 KB)Acknowledgments
This study was made possible through support by the Lundbeck Foundation, the Novo Nordisk Foundation, Aase og Ejnar Danielsens Fond, Agnes og Poul Friis Fond, Grosserer A.V. Lykfeldt og Hustrus Legat, Else og Mogens Wedell-Wedellsborgs Fond, Fonden af 17-12-1981, Kong Christian den Tiendes Fond and Frits, Georg og Marie Cecilie Gluds Legat. J.G.M. is a member of the Aarhus Research Center for Innate Immunology (ARCII) established through funding by the AU-Ideas program at Aarhus University.
Disclosure of Potential Conflicts of Interest
No potential conflicts of interest were disclosed.
References
- Bartel DP. MicroRNAs: genomics, biogenesis, mechanism, and function. Cell 2004; 116:281 - 97; http://dx.doi.org/10.1016/S0092-8674(04)00045-5; PMID: 14744438
- Lu J, Getz G, Miska EA, Alvarez-Saavedra E, Lamb J, Peck D, et al. MicroRNA expression profiles classify human cancers. Nature 2005; 435:834 - 8; http://dx.doi.org/10.1038/nature03702; PMID: 15944708
- McDermott AM, Heneghan HM, Miller N, Kerin MJ. The therapeutic potential of microRNAs: disease modulators and drug targets. Pharm Res 2011; 28:3016 - 29; http://dx.doi.org/10.1007/s11095-011-0550-2; PMID: 21818713
- Krützfeldt J, Rajewsky N, Braich R, Rajeev KG, Tuschl T, Manoharan M, et al. Silencing of microRNAs in vivo with ‘antagomirs’. Nature 2005; 438:685 - 9; http://dx.doi.org/10.1038/nature04303; PMID: 16258535
- Kay MA. State-of-the-art gene-based therapies: the road ahead. Nat Rev Genet 2011; 12:316 - 28; http://dx.doi.org/10.1038/nrg2971; PMID: 21468099
- Scherr M, Venturini L, Battmer K, Schaller-Schoenitz M, Schaefer D, Dallmann I, et al. Lentivirus-mediated antagomir expression for specific inhibition of miRNA function. Nucleic Acids Res 2007; 35:e149; http://dx.doi.org/10.1093/nar/gkm971; PMID: 18025036
- Primo MN, Bak RO, Schibler B, Mikkelsen JG. Regulation of pro-inflammatory cytokines TNFα and IL24 by microRNA-203 in primary keratinocytes. Cytokine 2012; 60:741 - 8; http://dx.doi.org/10.1016/j.cyto.2012.07.031; PMID: 22917968
- Gentner B, Schira G, Giustacchini A, Amendola M, Brown BD, Ponzoni M, et al. Stable knockdown of microRNA in vivo by lentiviral vectors. Nat Methods 2009; 6:63 - 6; http://dx.doi.org/10.1038/nmeth.1277; PMID: 19043411
- Haraguchi T, Ozaki Y, Iba H. Vectors expressing efficient RNA decoys achieve the long-term suppression of specific microRNA activity in mammalian cells. Nucleic Acids Res 2009; 37:e43; http://dx.doi.org/10.1093/nar/gkp040; PMID: 19223327
- Bak RO, Hollensen AK, Primo MN, Sørensen CD, Mikkelsen JG. Potent microRNA suppression by RNA Pol II-transcribed ‘Tough Decoy’ inhibitors. [Epub ahead of print] RNA 2012; http://dx.doi.org/10.1261/rna.034850.112; PMID: 23249752
- Haraguchi T, Nakano H, Tagawa T, Ohki T, Ueno Y, Yoshida T, et al. A potent 2′-O-methylated RNA-based microRNA inhibitor with unique secondary structures. Nucleic Acids Res 2012; 40:e58; http://dx.doi.org/10.1093/nar/gkr1317; PMID: 22259037
- Ebert MS, Neilson JR, Sharp PA. MicroRNA sponges: competitive inhibitors of small RNAs in mammalian cells. Nat Methods 2007; 4:721 - 6; http://dx.doi.org/10.1038/nmeth1079; PMID: 17694064
- Kluiver J, Gibcus JH, Hettinga C, Adema A, Richter MK, Halsema N, et al. Rapid generation of microRNA sponges for microRNA inhibition. PLoS One 2012; 7:e29275; http://dx.doi.org/10.1371/journal.pone.0029275; PMID: 22238599
- Sato K, Hamada M, Asai K, Mituyama T. CENTROIDFOLD: a web server for RNA secondary structure prediction. Nucleic Acids Res 2009; 37:Web Server issue W277-80; http://dx.doi.org/10.1093/nar/gkp367; PMID: 19435882
- Lanford RE, Hildebrandt-Eriksen ES, Petri A, Persson R, Lindow M, Munk ME, et al. Therapeutic silencing of microRNA-122 in primates with chronic hepatitis C virus infection. Science 2010; 327:198 - 201; http://dx.doi.org/10.1126/science.1178178; PMID: 19965718
- Wahid F, Shehzad A, Khan T, Kim YY. MicroRNAs: synthesis, mechanism, function, and recent clinical trials. Biochim Biophys Acta 2010; 1803:1231 - 43; http://dx.doi.org/10.1016/j.bbamcr.2010.06.013; PMID: 20619301
- Medina PP, Slack FJ. Inhibiting microRNA function in vivo. Nat Methods 2009; 6:37 - 8; http://dx.doi.org/10.1038/nmeth0109-37; PMID: 19116612
- Mikkelsen JG, Pedersen FS. Genetic reassortment and patch repair by recombination in retroviruses. J Biomed Sci 2000; 7:77 - 99; http://dx.doi.org/10.1007/BF02256615; PMID: 10754383
- Westerhout EM, Berkhout B. A systematic analysis of the effect of target RNA structure on RNA interference. Nucleic Acids Res 2007; 35:4322 - 30; http://dx.doi.org/10.1093/nar/gkm437; PMID: 17576691
- Bak RO, Stenderup K, Rosada C, Petersen LB, Moldt B, Dagnæs-Hansen F, et al. Targeting of human interleukin-12B by small hairpin RNAs in xenografted psoriatic skin. BMC Dermatol 2011; 11:5; http://dx.doi.org/10.1186/1471-5945-11-5; PMID: 21352568
- Jakobsen M, Stenderup K, Rosada C, Moldt B, Kamp S, Dam TN, et al. Amelioration of psoriasis by anti-TNF-alpha RNAi in the xenograft transplantation model. Mol Ther 2009; 17:1743 - 53; http://dx.doi.org/10.1038/mt.2009.141; PMID: 19568223
- Staunstrup NH, Madsen J, Primo MN, Li J, Liu Y, Kragh PM, et al. Development of transgenic cloned pig models of skin inflammation by DNA transposon-directed ectopic expression of human β1 and α2 integrin. PLoS One 2012; 7:e36658; http://dx.doi.org/10.1371/journal.pone.0036658; PMID: 22590584