Abstract
The conjugative transfer of F-like plasmids between bacteria is regulated by the plasmid-encoded RNA chaperone, FinO, which facilitates sense – antisense RNA interactions to regulate plasmid gene expression. FinO was thought to adopt a unique structure, however many putative homologs have been identified in microbial genomes and are considered members of the FinO_conjugation_repressor superfamily. We were interested in determining whether other members were also able to bind RNA and promote duplex formation, suggesting that this motif does indeed identify a putative RNA chaperone. We determined the crystal structure of the N. meningitidis MC58 protein NMB1681. It revealed striking similarity to FinO, with a conserved fold and a large, positively charged surface that could function in RNA interactions. Using assays developed to study FinO-FinP sRNA interactions, NMB1681, like FinO, bound tightly to FinP RNA stem-loops with short 5’ and 3’ single-stranded tails but not to ssRNA. It also was able to catalyze strand exchange between an RNA duplex and a complementary single-strand, and facilitated duplexing between complementary RNA hairpins. Finally, NMB1681 was able to rescue a finO deficiency and repress F plasmid conjugation. This study strongly suggests that NMB1681 is a FinO-like RNA chaperone that likely regulates gene expression through RNA-based mechanisms in N. meningitidis.
Introduction
In bacterial species, a multitude of small RNA species (sRNAs) are utilized to regulate gene expression, often through mechanisms in which the sRNA binds the Shine-Dalgarno sequence of the target mRNA to regulate translation.Citation1,Citation2 RNA chaperone proteins are commonly required to facilitate the binding of sRNAs to their mRNA partners. The best-studied example is Hfq, which regulates a number of genes in many Gram-positive and Gram-negative species.Citation3,Citation4
Bacteria have also evolved RNA chaperones that regulate gene activities in more specific circumstances. An example is FinO,Citation5 a protein expressed from the family of F-like plasmids, which serves to repress F plasmid gene expression in the absence of recipient cells through an antisense RNA-based mechanism that targets the mRNA of the H-NS de-silencing protein, TraJ.Citation6,Citation7 FinO binds the plasmid-encoded antisense sRNA, FinP,Citation8 protecting it from degradation by RNaseE,Citation9 and facilitating its interactions with traJ mRNA.Citation8–Citation12 This interaction is believed to sequester the Shine-Dalgarno sequence of the traJ message, and results in a 3–5-fold reduction in the transcription of TraJ-dependent transcripts and a 20–50-fold decrease in mating ability depending on the source of FinO.Citation13 The FinOP system appears to be widespread throughout F-like plasmids that have been linked to the acquisition of antibiotic resistance and virulence factors throughout a variety of human pathogens such as Salmonella type I strains.Citation14,Citation15
A series of biochemical and structural studies have provided detailed insights into the mechanism of FinO RNA chaperone activity. Stable stem-loop structures within both FinP and the traJ mRNA 5′ UTR provide a significant energy barrier against sense-antisense annealing. These stem-loop structures, with the adjacent 5′ and 3′ single-stranded tails, provide minimal, high affinity FinO binding sites.Citation16 The X-ray crystal structure of FinO revealed a unique, extended and largely helical fold, with flexible N- and C-terminal extensions.Citation10 While high resolution structural information for FinO-RNA complexes is lacking, site-specific protein-RNA crosslinking and FRET studies have indicated that a highly positively charged surface on one face of the FinO protein, as well as the extended N-terminal helix, contact minimal RNA targets.Citation17 Interestingly, whereas the core folded domain of FinO is sufficient for high-affinity interactions with stem-loop RNAs and protection of FinP against RNaseE, RNA chaperone activity depends upon the presence of the additional flexible N-terminal region.Citation18 The finding that deletion of this N-terminal region abolishes F plasmid conjugation repression strongly suggests that the RNA chaperone activity of FinO is essential for its role in the regulation of plasmid conjugation in vivo.Citation18
Unlike Hfq, FinO likely does not facilitate sense-antisense RNA pairing by simultaneously binding the two RNAs. Instead, FinO is thought to act through a destabilization of the base pairing within the stem of a single bound RNA. The reduction in the stability of internal base pairing is thought to reduce energy barriers to intermolecular pairing with the partner RNA. Support for this idea comes from the finding that FinO catalyzes the exchange of RNA strands between a two-stranded mimic of a high affinity RNA hairpin and a complementary single strand.Citation18 The initiation of duplexing between hairpin RNAs likely involves formation of a “kissing complex” between the complementary hairpin loops of FinP and traJ RNA,Citation11,Citation19 an interaction that may be stabilized by FinO.Citation10,Citation18
Here we demonstrate that the N. meningitidis protein, NMB1681, previously uncharacterized, is an RNA chaperone with structural and functional similarity to FinO. The crystal structure of NMB1681 reveals a structure with flexible N- and C-terminal tails and a central folded domain with a high degree of structural similarity to FinO. Using minimal FinO RNA targets, we demonstrate that NMB1681 binds RNA duplexes with 5′ and 3′ single-stranded tails but not single-stranded RNA. NMB1681 exhibits the key RNA chaperone activities of strand exchange and RNA duplexing and NMB1681, like FinO, can repress the conjugative transfer of F plasmid in vivo. We suggest that FinO-like proteins constitute a family of RNA chaperones that play highly specific roles in the regulation of bacterial gene expression.
Results
Crystal structure of N. meningitidis NMB1681 displays strong similarity to FinO.
BLAST analysis of the FinO protein revealed three major groups of homologs within the FinO conjugation repressor superfamily: FinO itself; the ProQ family involved in modulating proP expression; and a family of widely distributed hypothetical proteins found in the genus Neisseria as well as other genera that have fewer sequenced members (data not shown). Based on our previous studies on the FinO protein of plasmid F, we were interested in determining whether ProQ and the hypothetical protein in Neisseria were also capable of binding RNA and promoting duplex formation. ProQ will be discussed elsewhere (Chaulk et al. submitted); here, we investigate the ability of one hypothetical protein from Neisseria, NMB1681, to substitute for FinO in binding FinP sRNA and modulating F plasmid transfer frequency.
N. meningitidis is a gram-negative bacterium that infects the nasopharynx of 10–15% of the population and occasionally causes severe meningococcal disease.Citation20,Citation21N. meningitidis MC58 has been completely sequenced (Genbank AE002098).Citation22 However, very little is known about the post-transcriptional control of gene expression in this organism. Recently, the Hfq protein in MC58 was examined and was shown to be important for both the response to stress and virulence with a potentially large network of sRNAs regulating these traits.Citation23 NMB1681 is positioned downstream from a putative gene for chorismate synthase and in the opposite orientation to a neighboring gene for a putative topoisomerase IV subunit B also called ParE; this synteny appears to be conserved in the Neisseria species examined (data not shown). Unfortunately, the position of NMB1681 within the chromosome gives no clue to its function.
The structure of NMB1681 was determined by multi-wavelength anomalous dispersion (MAD) methods and refined to a resolution of 2.2 Å resolution ( and , Materials and Methods). A search of the protein structure database utilizing the DALI serverCitation24 revealed a high degree of structural similarity to the folded core of FinO (). Whereas NMB1681 exists as a monomer in solution as determined by gel filtration chromatography, the crystallographic asymmetric unit contains six NMB1681 chains, providing an opportunity to assess conservation of protein structure. Superposition of the six independent copies of the polypeptide reveals that the N-terminal 19 residues show significant conformational differences depending on their crystal packing environment (). In four of the six chains, the N-terminal helix (α1) is loosely packed against the body of the protein, whereas in one chain, it is directed away from the protein and is contiguous with α2. In another chain, this region is not visible in the electron density maps and we conclude that this region of the protein is likely disordered. Taken together, this suggests that the α1 helix is likely flexible in solution. The remainder of the protein shows little variation between the different chains. Structural conservation is most strong for residues 42–108 of NMB1681 and residues 94–164 of FinO, which align with an RMSD of 1.15 Å on 55 Cα atoms and a sequence identity of 24% (). The only significant structural difference within this region is at the C-terminus of α3, which is one turn longer in FinO than in NMB1681.
Previous protein-RNA crosslinking studies implicated the positively charged face on the main body of FinO, as well as the tip of the extended N-terminal helix (α1), as key RNA contacting regions.Citation17 The major positively charged surface on the main body of FinO is composed of residues from α4, two of which (Arg 66 and Arg 73) are conserved in NMB1681. The positively charged surface on NMB1681, however, wraps around to the opposite side of the protein, where residues from α2′, α5 and the β hairpin linking α5 and α6 all contribute to this surface ().
NMB1681 displays RNA chaperone activity.
The strong structural similarity of NMB1681 to FinO suggests that NMB1681 may also function as an RNA chaperone. To test this hypothesis, RNA binding, RNA strand exchange and RNA duplexing assays were carried out with NMB1681, using minimal high affinity FinO RNA substrates based on FinP stem-loop II (SLII) and the complementary region in traJ mRNA. These RNAs contain a 14 base pair stem, 7 nucleotide loop and short 5′ and 3′ single-stranded tails. In addition, a two-stranded SLII mimic, SII, which lacks the SLII loop region but is bound by FinO with the same affinity as SLII, was also utilized in these experiments.
Electrophoretic mobility shift assays showed that NMB1681 binds SII with high affinity, similar to the affinity of FinOCitation18 for this RNA (Kd = 36 ± 4 nM, ). Like FinO, this binding was specific to the double-stranded form of SII, as the single-stranded RNA, SII(A) was bound only very weakly by NMB1681 under these conditions.
We utilized an RNA stand exchange assay to test the RNA chaperone activity of NMB1681. In this assay, one strand of the SII duplex (SII(A)) is labeled with 32P and is challenged with an excess of an unlabelled version of SII(A). Conversion of the double stranded form of SII(A) to single strand is monitored by native gel electrophoresis (). NMB1681 displayed some RNA strand exchange activity, although significantly less than FinO ( and C). Because NMB1681 binds SII duplex but not the single strand with high affinity, we suggest that NMB1681 destabilizes the duplex form of SII, thereby reducing the free energy barrier to strand exchange.
Finally we tested the ability of NMB1681 to catalyze duplex formation between FinP SLII and a complementary RNA, SLIIc from traJ mRNA (). Due to the high stabilities of the internal stem-loop structures, these RNAs show essentially no duplexing during a 120 min. reaction at 37°C, however, NMB1681 facilitates a dramatic increase in RNA duplexing activity using the SLII and SLIIc hairpin substrates, similar to the levels achieved with FinO ( and C). Thus, NMB1681 constitutes an RNA chaperone with similar RNA binding and chaperone activities as FinO.
NMB1681 rescues finO to repress F plasmid conjugation.
The demonstrated RNA binding and RNA chaperone activities of NMB1681 on FinO RNA substrates, derived from FinP, suggested that NMB1681 might rescue a finO deficiency to regulate F plasmid conjugation. To test this, we used an F derivative, pOX38-Km, which does not express FinO and therefore conjugates with high efficiency. To test the ability of NMB1681 to repress conjugation of this plasmid, NMB1681 or FinO proteins were coexpressed from a second plasmid, pBAD24, in the same cells as pOX38-Km. These cells were mixed with donor cells and mating efficiencies were calculated as the ratios of transconjugants to donor cells (Materials and Methods).
As found previously, conjugation of pOX38-Km was inhibited ∼100-fold by expression of FinO from pBAD24 (). Deletion of the N-terminal 44 residues of FinO, which are essential for RNA chaperone function but not RNA binding, completely abolished this repression, whereas a shorter deletion of 25 residues did not impact repression of conjugation. NMB1681 repressed conjugation ∼10-fold, suggesting that this protein can partially rescue FinO function. We also asked if the flexible N-terminal region of NMB1681 might also be important for conjugative repression. We therefore cloned and expressed NMB1681Δ, which lacks the N-terminal 17 residues corresponding to the flexible α1 helix. This protein was also able to repress conjugation ∼10-fold, demonstrating that this region has no role in the repression of conjugation.
FinO not only directly facilitates FinP—traJ sense-antisense RNA pairing, but it also binds and protects FinP from RNaseE degradation, which likely is also important for plasmid repression. To assess the ability of NMB1681 to protect FinP, we compared the abundance of FinP RNA at 0 and 120 min, after rifampicin-induced blockage of transcription, between cells expressing FinO, NMB1681 or a control protein that does not regulate FinP, TraD (). Consistent with previous results,Citation18 FinP levels were significantly reduced such that ∼90% of the initial FinP transcripts were degraded after 120 min. in cells expressing the control protein, TraD. In contrast, expression of either FinO, FinO26–186 or FinO45–186, effectively protected FinP such that only ∼10–20% of FinP transcripts were degraded after 120 min rifampicin treatment. Expression of either NMB1681 or the N-terminal deletion mutant, NMB1681Δ, resulted in weak protection of FinP where ∼80% of initial FinP transcripts were degraded after 120 min. rifampicin treatment, corresponding to a ∼2-fold protection compared to the TraD control. Interestingly, like FinO but not its N-terminal deletion derivatives, the levels of FinP in cells expressing NMB1681 or NMB1681Δ at 0 minutes were elevated compared to the negative TraD control. This suggests that FinP could accumulate in cells containing NMB1681 due to this weak protection from degradation. This slower accumulation coupled with a reduced ability to catalyze duplex formation might explain the corresponding reduction in the ability of NMB1681 to repress conjugation compared to FinO.
Discussion
Whereas it is generally accepted that RNA-RNA interactions are facilitated by diverse RNA chaperones and ATP-dependent RNA helicases, unifying themes that explain the mechanisms that underlie these activities are still poorly understood. Here we show that the N. meningitidis protein, NMB1681, which was identified as belonging to the FinO conjugation_repressor superfamily by in silico analysis, has RNA chaperone activity and could be important in modulating RNA-RNA interactions in Neisseria.
FinO-like domains provide an RNA-binding anchor for RNA chaperones.
Detailed biochemical dissection of the FinO chaperone function showed that the region most critical for RNA binding, the folded core domain, is distinct from the region essential for chaperone function, the flexible N-terminal region.Citation18 The N-terminal region corresponding to residues 1–61, shows little detectable RNA binding in EMSAs, but nevertheless shows significant strand exchange and duplexing activities. Intriguingly, deletion or missense mutations within this N-terminal region which compromise chaperone function resulted in significantly enhanced RNA binding affinity. This result provided support for the suggestion that the free energy required to destabilize intramolecular RNA secondary structures might be provided by RNA binding interactions.Citation18 Flexible regions are commonly found in RNA chaperones and an “entropy transfer” model has been proposed whereby protein disorder is transferred to a bound RNA substrate to overcome energy barriers within kinetically trapped RNA substrates.Citation25 While NMB1681 displays high levels of RNA duplexing similar to FinO, its RNA strand exchange activity appears to be significantly lower, which may due to differences in the structure and flexibilities of the N-terminal regions in these two proteins.
NMB1681 structurally resembles FinO, with a shorter N-terminal flexible extension. The presence of non-crystallographic symmetry in the NMB1681 structure provides a view of different conformations that can be accessed by this N-terminal region. In five of the six copies in the asymmetric unit, this extension adopts a helical conformation in a variety of orientations that accommodate the different crystal packing environments. The region of the FinO N-terminal region that is visualized in its crystal structure is also helical, but unlike the majority of the NMB1681 chains, this extension extends away from the main body of the protein, and this orientation is probably stabilized through interactions with the C-terminal α6 helix (). The apparently rigid, extended form of the FinO N-terminal helix observed in the crystal structure is likely misleading and this region is probably quite flexible. This helix, as well as part of the C-terminal helix, is more sensitive to proteolysis than the central folded domain, corresponding to residues 62–174,Citation26 and an analysis of the crystallographic B factors also indicates that the N- and C-terminal regions are more flexible than the folded core.Citation10 These regions in FinO may undergo a reorientation as they interact with RNA as FRET studies reveal that both the N-terminus of FinO α1, as well as residues in α4, come into close contact with the 5′-single-stranded tail of a bound SLII.Citation17 While NMB1681 displays a striking structural similarity to FinO, differences in its electrostatic potential surface, especially the presence of a significant positively charged surface in NMB1681 that is not present in FinO ( and bottom part), suggest that the details of NMB1681-RNA interactions may differ from those of FinO. High resolution structural studies of FinO- and NMB1681-RNA complexes will be required to address these issues.
Our finding that NMB1681 has structural and functional similarities to the well-characterized E. coli RNA chaperone, FinO, strongly suggests that this protein functions to modulate sRNA-mediated processes in Neisseria. sRNA transactions are likely critical for Neisseria pathogenicity, as the Neisseria Hfq homolog is a major virulence factor in this organism.Citation23 Whereas Hfq chaperones act in a relatively non-specific manner, FinO appears to target only the FinP-traJ mRNA pair, but not other sRNAs. It is therefore possible that NMB1681 may also act in a highly selective manner. Horizontal gene transfer is common in Neisseria species and the conjugative transfer of plasmids in particular have been linked to antibiotic resistance in N. gonorrhea.Citation27 NMB1681 is situated near the boundary of a region of the genome that arose from horizontal transfer within N. meningitidis,Citation22 thus it is possible that this protein may, like FinO, have been acquired from a gene pool shared by N. meningitidis and E. coli.
Materials and Methods
Protein cloning, expression and purification.
The gene encoding the protein NMB1681 of Neisseria meningitidis MC58 was cloned into pMCSG7 vector.Citation28 The protocols for the expression in E. coli BL21 (DE3) and purification of the SeMet-labeled recombinant protein were reported previously.Citation29 The NMB1681 was expressed well with final protein yield of about 50 mg/L culture. For mating assays and FinP stability studies, genes encoding NMB1681, NMB1681Δ (encoding residues 18–141), FinO or the N-terminal FinO deletion mutants, FinO26–186 and FinO45–186, were cloned into pBAD24.
Protein crystallization.
The Se-Met labeled NMB1681 protein was screened for crystallization conditions with the help of the Mosquito robot (TTP Labtech) using the sitting drop vapor diffusion technique in 96-well CrystalQuick plates (Greiner). For each condition, 0.4 µL of protein (∼91 mg/mL) and 0.4 µL of crystallization formulation were mixed; the mixture was equilibrated against 135 µL of the reservoir in the well. Initial crystallization screens used were Index (Hampton Research) and Wizard (Emerald Biosystems) at both 4°C and 18°C. Diffraction quality crystals of NMB1681 appeared under the optimized condition of 0.1 M Bis-tris, pH 6.5, 0.17 M MgCl2, 21% (w/v) PEG3350. Prior to data collection, crystals were treated with cryoprotectant (15% glycerol) added to crystallization buffer.
X-ray diffraction data collection.
Diffraction data were collected at 100 K at the 19ID beamline of the Structural Biology Center at the Advanced Photon Source at Argonne National Laboratory using the program SBCcollect. A single-wavelength anomalous diffraction (SAD) data set was collected at the wavelength near selenium absorption peak from one Se-Met labeled protein crystal of NMB1681. All data were processed and scaled with HKL3000 suiteCitation30 ().
Structure determination and refinement.
The NMB1681 crystal structure was determined using SAD method. Se sites were first located using the program SHELXDCitation31 and they were used for initial phasing with the program MLPHARE (CCP4 suite; http://www.ccp4.ac.uk/). After density modification (DM; CCP4), partial models of 6 monomers (455 residues in total with 306 side chains placed) were obtained from automatic model building trials using the program ARP/wARP.Citation32 All the above programs are integrated within the program suite HKL3000.Citation30 After cycles of model buildings using the program COOT,Citation33 the models were refined using the program PhenixCitation34 (). After final refinements, the electron density map calculated at 1.0 σ was well connected throughout the main chains of the six NMB1681 monomers. The electron density for the N-terminal region (M1-S17) of one of the monomers is missing. The density for the C-terminal region (A125-E141) is missing for all six monomers. The structural factors and coordinates of NMB1681 structure are available in the PDB data bank under the access code 3MW6.
Overexpression and purification of NMB1681 for functional assays.
Cells harboring the relevant expression vectorCitation29 were grown to OD600 of ∼1, then brought to 18°C and induced with 0.6 mM IPTG (isopropyl-β-D-thiogalactopyranoside) overnight. Cell pellets were resuspended in lysis buffer (50 mM Hepes (pH 7.3), 500 mM NaCl, 1 mM DTT, 5% (v/v) glycerol, 1 mM EDTA) containing protease inhibitor tablets (Roche, as per manufacturer's instructions) and lysed by sonification. Cleared lysates were purified by Ni(NTA) affinity chromatography (20 mM to 250 mM imidazole gradient in lysis buffer) and size exclusion chromatography using lysis buffer.
RNA preparation.
RNAs were prepared by in vitro run-off transcription reactions using bacteriophage T7 RNA polymerase with the appropriate DNA template and complementary primer. The primer binding sites are underlined in the following sequences.
SLII. 5′-GGA CTC GCC GAT GCA GGG AGA CGT GAA CTC CCT GCA TCG ACT GTC CTATAGTGAGTCGTATTA-3′
SLIIc. 5′-GGA CAG TCG ATG CAG GGA GTT CAC GTC TCC CTG CAT CGG CGA GTC CTATAGTGAGTCGTATTA-3′
SII(A). 5′-GGT CCT GCA TCG ACT GTC CTATAGTGAGTCGTATTA-3′
SII(B). 5′-AAA ATC GCC GAT GCA GGA CCTATAGTGAGTCGTATTA-3′.
RNA binding electrophoretic mobility shift assay (EMSA).
Binding experiments were formed on ice in 100 µL reaction mixtures based on binding reaction buffer (25 mM Tris-HCl pH 7.5, 100 mM NaCl, 1 mM EDTA), containing ∼50,000 cpm of 32P 5′ end-labeled SIIA or 32P 5′ end-labeled SIIA duplexed with an equimolar amount of unlabeled SIIB. Prior to their addition, SLIIA or SLIIA/B were heat denatured at 90°C in binding reaction buffer and cooled slowly to room temperature (2°C/min). Reaction mixtures were incubated for 20 min, on ice, before 10 µL of 20% glycerol was added and subjected to 6% (19:1) non-denaturing PAGE (190 mM Glycine, 25 mM Tris, pH 8, 2–3 h at 180 V). The gel was exposed overnight with a phosphor screen (GE Healthcare). ImageQuant software was used to visualize the gel. Gels were exposed to Molecular Dynamics storage phosphor screen and scanned using a Storm 840 phosphorimager (Molecular Dynamics). Bands were quantified with ImageQuanNT software (Molecular Dynamics) and binding affinities were obtained using GraphPad PRISM 5.02. Standard deviations for the binding affinities were calculated based on three independent experiments.
RNA strand exchange assay.
Strand exchange assays were performed as previously describedCitation18 on ice in 12 µL reaction mixtures containing 25 mM Tris (pH 8.1), 50 µg/mL bovine serum albumin (BSA), 5% (v/v) glycerol, 0.05% (v/v) β-mercaptoethanol and 10 mM NaCl, 2 units (1 µL) of RNAguard (Amersham), 10 µM protein, 5 nmoles of the labeled SIIA/B duplex (described above) to give a final duplex concentration of ∼400 pM and 2.5 µmoles of the unlabeled SIIA strand (to give a final concentration of ∼200 nM). Strand exchange assays were initiated by placing reaction tubes at 37°C and stopped at the indicated times by adding an equal volume of stop solution (5% (v/v) glycerol, 0.4% (w/v) SDS and 20 mM EDTA). Samples were subjected to 15% (19:1) non-denaturing PAGE (190 mM Glycine, 25 mM Tris, pH 8, 180 V for 2 h) at room temperature to separate the free and duplexed 32P-labeled strands. Gels were exposed and analyzed as described above.
RNA duplexing assays.
Duplexing between SLII and SLIIc was measured as previously describedCitation18 using 96 µL reaction mixtures containing 25 mM Tris (pH 8.1), 50 µg/mL BSA, 5% (v/v) glycerol, 0.05% (v/v) β-mercaptoethanol, 20 mM NaCl, 10 µM protein, 1 µM SLIIc and 50 nM 32P-labeled SLII. To initiate duplexing, labeled SLII, preincubated at 37°C, was added to reaction mixtures, also preincubated at 37°C and lacking SLII. 12 µL aliquots were taken at various time points and added to 12 µL of stop solution (as above). Samples were subjected to 10% (19:1) non-denaturing PAGE (190 mM Glycine, 25 mM Tris, pH 8, 180 V 2 h) prior to visualization and quantification of the labeled SLII as described above.
Northern blot analysis of FinP stability.
The stability of FinP RNA isolated from bacterial cultures used in the mating assays was assessed by northern blot analysis essentially as previously described.Citation9,Citation12,Citation18 2 µg total RNA (isolated with Trizol (from 1 mL of bacterial culture) were loaded per lane and gels (15% (19:1) 8 M urea) were transferred on GeneScreen PlusR Hybridization Transfer Membrane (Perkin Elmer). Blots were first probed with a 5′ 32P end labeled FinP anti-sense DNA probe, stripped and re-probed with 5′ 32P end-labeled tRNAser anti-sense DNA probe. ULTRAhyb-Oligo (Ambion) was used as hybridization buffer, 1 × 106 cpm of probe per mL hybridization buffer was used for probing. Hybridization and washing were performed at 42°C. FinP intensities (ImageQuant) were normalized to tRNAser intensities and comparisons between 0 and 120 minutes were made to quantify relative FinP stability.
Mating assays.
Donor XK1200 cells and ED24 recipient cells were grown to mid-exponential phase at 37°C, with arabinose added to 0.05% to donor cells for induction of 1 hour prior to mating. Mating assays were performed as previously described.Citation36 Mating efficiency is calculated as the number of transconjugants divided by the number of donors. Each assay was repeated 3 times and the averaged values are reported.
Figures and Tables
Figure 1 X-ray crystal structure of NMB1681 reveals structural similarity to FinO. (A) Stereo view of an alignment of the six NMB1681 polypeptide chains in the crystallographic asymmetric unit. Alpha helices are numbered in accordance with the FinO numbering system. (B) Comparison of the structures of FinO (orange ribbon) and NMB1681 (blue ribbon) from two different orientations. Images in the top part are in the same orientation as in (A) whereas those in the bottom part are related to those in the top by a 180° rotation about the vertical axis. In each part, the left image shows a structural alignment of NMB1681 with FinO. Chain termini and secondary structure elements are labeled, as is residue 45 in FinO. Truncation of the region N-terminal to residue 45 does not reduce RNA binding activity but abolishes RNA strand exchange and duplexing activities. The middle and right images show the electrostatic surfaces of FinO (middle) and NMB1681 (right). (C) Structure-based sequence alignment of FinO, ProQ and NMB1681 with secondary structures indicated. Identical residues or residues with conserved charge are highlighted (orange, identical hydrophobic residues; red, conserved negatively charged residues; blue, conserved positively charged residues; green, identical hydrophilic residues).
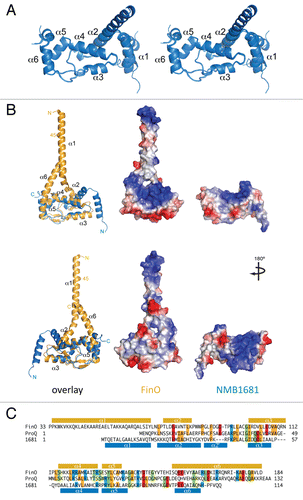
Figure 2 RNA binding activity of NMB1681. (A) Electrophoretic gel mobility shift assays (EMSA) were used to assess interactions between NMB1681 and a double stranded RNA (SIIA/B, left part) or a single-stranded RNA (SIIA, right part). (B) Quantitation of EMSA results for the binding of NMB1681 to double stranded RNA (dsRNA). Fitting of the binding curves suggest NMB1681 binds dsRNA with a Kd of 36 ± 4 nM.
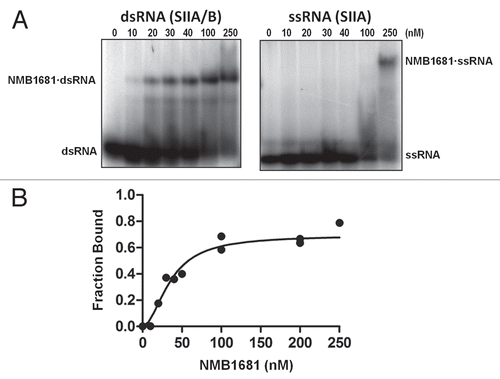
Figure 3 RNA strand exchange activity of NMB1681. (A) Schematic diagram of the strand exchange assay. A two-stranded mimic of FinP SLII (SIIA/B) in which the (A) strand is labeled with 32P (*), is challenged with an excess of unlabeled SIIA. Exchange of the labeled SIIA from duplex to single strand is monitored by gel electrophoresis. (B) Strand exchange of NMB1681 was compared to FinO or a no protein control over a 180 min. time course utilizing continuous native gel electrophoresis. The positions of the duplex and single stranded species are indicated, as well as degradation species (*). (C) Using data from (B) (averaged from two independent experiments), the fraction of exchanged (released) SIIA was fit to a first order exponential giving rates of strand exchange of 0.032 ± 0.007 min−1 and 0.011 ± 0.004 min−1 for FinO and NMB1681 respectively.
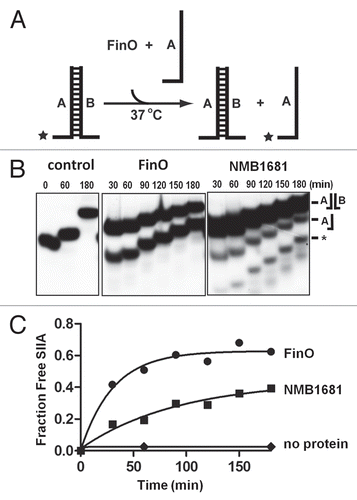
Figure 4 RNA annealing activity of NMB1681. (A) Schematic diagram of the RNA duplexing assay. Stem-loop II from FinP (SLII) is labeled with 32P (*) was incubated with an excess of unlabeled, complementary SLIIc from traJ mRNA. Duplex formation between these RNAs is monitored as a function of time by native gel electrophoresis. (B) Duplex formation between SLII and SLIIc RNAs is monitored over a 120 min. time course by continuous native gel electrophoresis, catalyzed by NMB1681, FinO or no protein control, as indicated. The positions of the hairpin and duplex forms of the RNAs are indicated as well as the position of an RNA degradation product (*). (C) Using data from (B) (from two independent experiments), the fraction of duplex formed was fit to a first order exponential giving rates of duplexing of 0.13 ± 0.03 min−1 and 0.21 ± 0.05 min−1 for FinO and NMB1681 respectively.
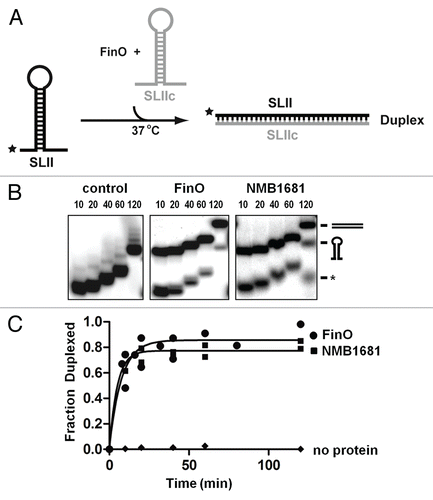
Figure 5 NMB1681 exhibits weak protection of FinP in vivo. FinP stability in E. coli cells was assessed at 0 and 120 min. following rifampicin treatment by northern blot analysis. FinP stabilities were compared between cells expressing either NMB1681, the N-terminal deletion mutant, NMB1681Δ, full length FinO or two N-terminal deletion mutants (FinO26–186 and FinO45–186), the control protein, TraD or the pBAD24 vector. The fraction of FinP protected at 120 min. post-rifampicin compared to the 0 min. time point was calculated after normalization to the tRNASer loading control.
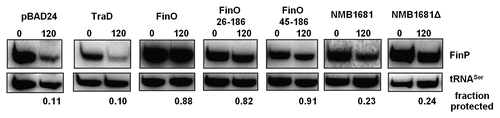
Table 1 Crystallographic statistics
Table 2 Fertility inhibition of the F plasmid (pOX38-Km) by FinO or NMB1681 constructs
Acknowledgements
The authors wish to thank members of Structural Biology Center at Argonne National Laboratory for their help with data collection at the 19ID beamline. We also thank Min Zhou, Erika Duggan and James Abdullah for helps in cloning, protein expression, purification and crystallization. This work was supported by a grant from the Canadian Institutes of Health Research (CIHR) to J.N.M.G. and National Institutes of Health grant GM074942 and by the US Department of Energy, Office of Biological and Environmental Research, under contract DE-AC02-06CH11357 to A.J. J.N.M.G. acknowledges the support of the Howard Hughes Medical Institute International Scholar program.
References
- Gottesman S. The small RNA regulators of Escherichia coli: roles and mechanisms*. Annu Rev Microbiol 2004; 58:303 - 328
- Majdalani N, Vanderpool CK, Gottesman S. Bacterial small RNA regulators. Crit Rev Biochem Mol Biol 2005; 40:93 - 113
- Brennan RG, Link TM. Hfq structure, function and ligand binding. Curr Opin Microbiol 2007; 10:125 - 133
- Valentin-Hansen P, Eriksen M, Udesen C. The bacterial Sm-like protein Hfq: a key player in RNA transactions. Mol Microbiol 2004; 51:1525 - 1533
- Finnegan D, Willetts N. The nature of the transfer inhibitor of several F-like plasmids. Mol Gen Genet 1972; 119:57 - 66
- Will WR, Frost LS. Hfq is a regulator of F-plasmid TraJ and TraM synthesis in Escherichia coli. J Bacteriol 2006; 188:124 - 131
- Frost LS, Koraimann G. Regulation of bacterial conjugation: balancing opportunity with adversity. Future Microbiol 2010; 5:1057 - 1071
- van Biesen T, Frost L. The FinO protein of IncF plasmids binds FinP antisense RNA and its target, traJ mRNA and promotes duplex formation. Mol Microbiol 1994; 14:427 - 436
- Jerome L, van Biesen T, Frost L. Degradation of FinP antisense RNA from F-like plasmids: the RNA-binding protein, FinO, protects FinP from ribonuclease E. J Mol Biol 1999; 285:1457 - 1473
- Ghetu A, Gubbins M, Frost L, Glover J. Crystal structure of the bacterial conjugation repressor finO. Nat Struct Biol 2000; 7:565 - 569
- Gubbins M, Arthur D, Ghetu A, Glover J, Frost L. Characterizing the structural features of RNA/RNA interactions of the F-plasmid FinOP fertility inhibition system. J Biol Chem 2003; 278:27663 - 27671
- Sandercock J, Frost L. Analysis of the major domains of the F fertility inhibition protein, FinO. Mol Gen Genet 1998; 259:622 - 629
- Lee S, Frost L, Paranchych W. FinOP repression of the F plasmid involves extension of the half-life of FinP antisense RNA by FinO. Mol Gen Genet 1992; 235:131 - 139
- Boyd E, Hartl D. Recent horizontal transmission of plasmids between natural populations of Escherichia coli and Salmonella enterica. J Bacteriol 1997; 179:1622 - 1627
- Boyd E, Hartl D. Salmonella virulence plasmid. Modular acquisition of the spv virulence region by an F-plasmid in Salmonella enterica subspecies I and insertion into the chromosome of subspecies II, IIIa, IV and VII isolates. Genetics 1998; 149:1183 - 1190
- Jerome L, Frost L. In vitro analysis of the interaction between the FinO protein and FinP antisense RNA of F-like conjugative plasmids. J Biol Chem 1999; 274:10356 - 10362
- Ghetu A, Arthur D, Kerppola T, Glover J. Probing FinO-FinP RNA interactions by site-directed protein-RNA crosslinking and gelFRET. Rna 2002; 8:816 - 823
- Arthur D, Ghetu A, Gubbins M, Edwards R, Frost L, Glover J. FinO is an RNA chaperone that facilitates sense-antisense RNA interactions. EMBO J 2003; 22:6346 - 6355
- Koraimann G, Teferle K, Markolin G, Woger W, Hogenauer G. The FinOP repressor system of plasmid R1: analysis of the antisense RNA control of traJ expression and conjugative DNA transfer. Mol Microbiol 1996; 21:811 - 821
- Rosenstein NE, Perkins BA, Stephens DS, Popovic T, Hughes JM. Meningococcal disease. N Engl J Med 2001; 344:1378 - 1388
- Tinsley C, Nassif X. Meningococcal pathogenesis: at the boundary between the pre- and post-genomic eras. Curr Opin Microbiol 2001; 4:47 - 52
- Tettelin H, Saunders NJ, Heidelberg J, Jeffries AC, Nelson KE, Eisen JA, et al. Complete genome sequence of Neisseria meningitidis serogroup B strain MC58. Science 2000; 287:1809 - 1815
- Fantappie L, Metruccio MM, Seib KL, Oriente F, Cartocci E, Ferlicca F, et al. The RNA chaperone Hfq is involved in stress response and virulence in Neisseria meningitidis and is a pleiotropic regulator of protein expression. Infect Immun 2009; 77:1842 - 1853
- Holm L, Kaariainen S, Rosenstrom P, Schenkel A. Searching protein structure databases with DaliLite v.3. Bioinformatics 2008; 24:2780 - 2781
- Tompa P, Csermely P. The role of structural disorder in the function of RNA and protein chaperones. FASEB J 2004; 18:1169 - 1175
- Ghetu AF, Gubbins MJ, Oikawa K, Kay CM, Frost LS, Glover JN. The FinO repressor of bacterial conjugation contains two RNA binding regions. Biochemistry 1999; 38:14036 - 14044
- Pachulec E, van der Does C. Conjugative plasmids of Neisseria gonorrhoeae. PLoS One 2010; 5:9962
- Stols L, Gu M, Dieckman L, Raffen R, Collart FR, Donnelly MI. A new vector for high-throughput, ligation-independent cloning encoding a tobacco etch virus protease cleavage site. Protein Expr Purif 2002; 25:8 - 15
- Kim Y, Dementieva I, Zhou M, Wu R, Lezondra L, Quartey P, et al. Automation of protein purification for structural genomics. J Struct Funct Genomics 2004; 5:111 - 118
- Minor W, Cymborowski M, Otwinowski Z, Chruszcz M. HKL-3000: the integration of data reduction and structure solution—from diffraction images to an initial model in minutes. Acta Crystallogr D Biol Crystallogr 2006; 62:859 - 866
- Schneider TR, Sheldrick GM. Substructure solution with SHELXD. Acta Crystallogr D Biol Crystallogr 2002; 58:1772 - 1779
- Cohen SX, Morris RJ, Fernandez FJ, Ben Jelloul M, Kakaris M, Parthasarathy V, et al. Towards complete validated models in the next generation of ARP/wARP. Acta Crystallogr D Biol Crystallogr 2004; 60:2222 - 2229
- Emsley P, Cowtan K. Coot: model-building tools for molecular graphics. Acta Crystallogr D Biol Crystallogr 2004; 60:2126 - 2132
- Adams PD, Afonine PV, Bunkoczi G, Chen VB, Davis IW, Echols N, et al. PHENIX: a comprehensive Python-based system for macromolecular structure solution. Acta Crystallogr D Biol Crystallogr 2010; 66:213 - 221
- Smith M, Kwok S, Hodges R, Wood J. Structural and functional analysis of ProQ: an osmoregulatory protein of Escherichia coli. Biochemistry 2007; 46:3084 - 3095
- Lu J, Manchak J, Klimke W, Davidson C, Firth N, Skurray RA, et al. Analysis and characterization of the IncFV plasmid pED208 transfer region. Plasmid 2002; 48:24 - 37