Figures & data
Table 1 Design specification of the 100-MWth prismatic HTGR
Table 2 The properties of burnable poisons [Citation6,Citation11]
Figure 4 Microscopic absorption cross section of main absorbing isotopes [Citation11]
![Figure 4 Microscopic absorption cross section of main absorbing isotopes [Citation11]](/cms/asset/d6503a43-fe35-4581-98b5-845ed66e36a8/tnst_a_815138_o_f0004g.jpg)
Figure 5 The sensitivity of the parameters obtained using the combination of B4C + Gd2O3 particles loading
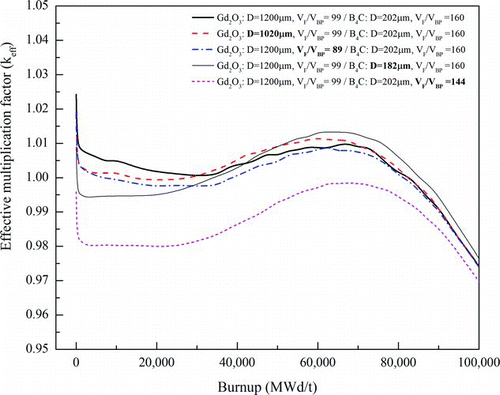
Table 3 Summary of the burnup characteristics of a reactor without BP particles and with the appropriate loading of BP particles
Figure 6 The effective multiplication factor (k eff) of the reactor without and with BP particles insertion at 1200 K
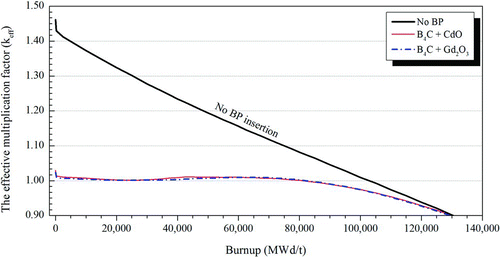
Table 4 The change in reactivity achieved by decreasing the parameters by 1% in the appropriate loading of B4C + Gd2O3 particles
Figure 7 The change in the k eff by decreasing the diameter and the V F/V BP by 1% for the appropriate loading of B4C + Gd2O3 particles
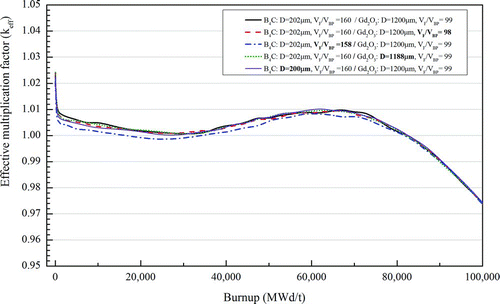
Table 5 Summary of the burnup characteristics of a reactor without BP particles and with the appropriate loading of BP particles to avoid a prompt supercritical accident
Figure 8 Change in the number densities of 239 Pu, 240Pu, 241Pu, and 242Pu isotopes in the fuel kernels for the appropriate loading of B4C + Gd2O3 particles
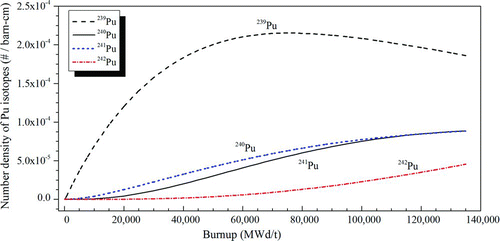
Figure 9 Change in the number densities of 155Gd and 157Gd in Gd2O3 particles and 10B in B4C particles for the appropriate loading of B4C + Gd2O3 particles
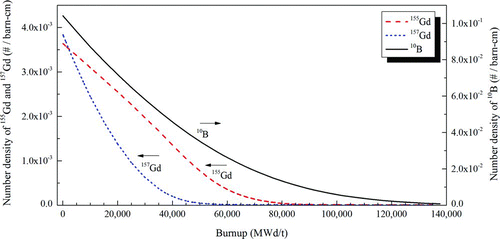
Figure 10 Change in the number densities of 113Cd in CdO particles and 10B in B4C particles for the loading of B4C + CdO particles
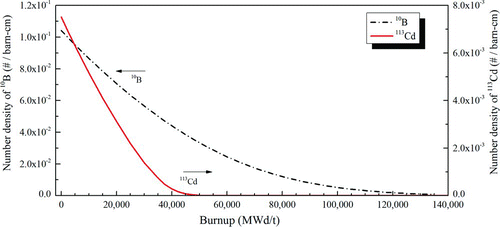
Figure 11 The change in the k eff when the core temperature decreases from 1200 K for the appropriate loading of B4C + Gd2O3 particles
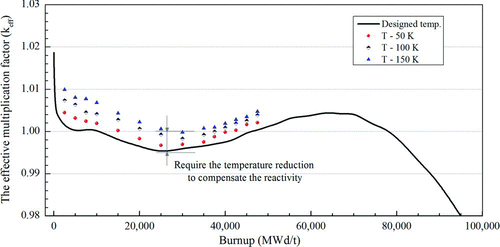
Figure 12 The change in the k eff when the core temperature decreases from 1200 K for the appropriate loading of B4C + CdO particles
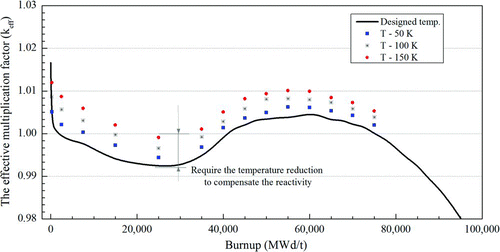
Table 6 Compensation for the reactivity worth by decreasing the core temperature for reactors with the appropriate loading of BP particles to avoid a prompt supercritical accident
Figure 13 The neutron flux in fuel kernels at energy level 0.01–100 eV in various burnup periods: 10 GWd/t, 30 GWd/t, and 42.5 GWd/t
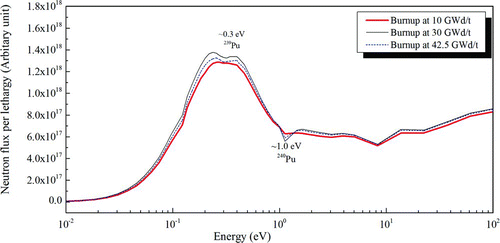
Figure 14 Diagram of the direct Brayton cycle [Citation12]
![Figure 14 Diagram of the direct Brayton cycle [Citation12]](/cms/asset/249b8a51-fba8-465d-abcb-ced5877b424d/tnst_a_815138_o_f0014g.gif)
Figure 15 The thermal efficiency of a gas turbine direct Brayton cycle in GT-MHR as a function of turbine inlet temperature [Citation13]
![Figure 15 The thermal efficiency of a gas turbine direct Brayton cycle in GT-MHR as a function of turbine inlet temperature [Citation13]](/cms/asset/4b9ba2d0-46be-4e45-bbe0-39a782260735/tnst_a_815138_o_f0015g.jpg)
Table 7 Summary of the burnup characteristics of a reactor without BP particles and with the appropriate loading of BP particles to avoid any supercritical accident during operation
Table 8 Compensation for the reactivity worth by decreasing the core temperature for subcritical reactors with the appropriate loading of BP particles to avoid any supercritical accident during operation
Figure 16 The change in the k eff when the core temperature decreases from 1200 K for the appropriate loading of B4C + Gd2O3 particles
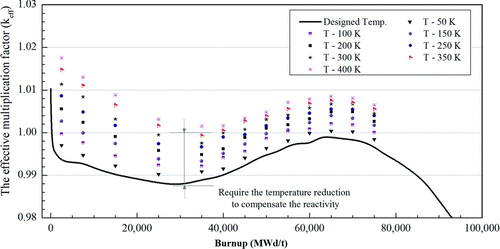
Figure 17 The change in the k eff when the core temperature decreases from 1200 K for the appropriate loading of B4C + CdO particles
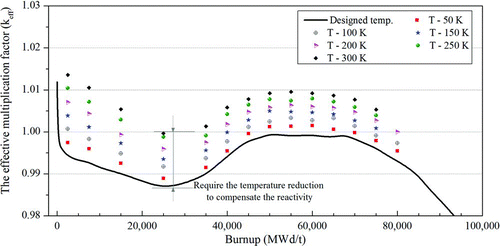