Figures & data
Table 1. The vibration frequencies (ω in unit of THz) of H at the TS (ωTS) and OS (ωOS) as well as transition states (ωTran) in W and W-based alloy at 0 K
Table 2. The ratio (aT/a0) of lattice parameter, the lattice parameter (aT) and the lattice expansion strain (%) of W-based alloy at the relevant temperature
Figure 1. (Top view from the [001] direction) The different neighbor TS (a) and OS (b) around the substitute Cr/V (gray ball) in W (red ball) atomic structure plane supercell. The distances of 1nn, 2nn, 3nn, 4nn TS (OS) are (a/2),
(
),
(
), and
(3a/2), respectively. The TS and OS are denoted by triangle and circle, respectively.
![Figure 1. (Top view from the [001] direction) The different neighbor TS (a) and OS (b) around the substitute Cr/V (gray ball) in W (red ball) atomic structure plane supercell. The distances of 1nn, 2nn, 3nn, 4nn TS (OS) are 5a/4(a/2), 13a/4(2a/2), 29a/4(5a/2), and37a/4(3a/2), respectively. The TS and OS are denoted by triangle and circle, respectively.](/cms/asset/dd14d9c0-202f-4823-9d18-798fddd3e59d/tnst_a_1383210_f0001_oc.jpg)
Figure 2. The formation energies of H at the TS with the increasing temperature in W and W-based alloys. (a) The total formation energy in reference to the total H chemical potential μH(T, P1atm) = μH(T = 0 K) + μH(T ≠ 0 K, P1atm). (b) The corresponding static formation energy in reference to the static H chemical potential μH(T = 0 K) based on EquationEquation (13)(13)
(13) and it is related to the lattice expansion. (c) Phonon vibration formation energy referring to the temperature-dependent H chemical potential μH(T ≠ 0 K, P1atm) based on EquationEquation (14)
(14)
(14) .
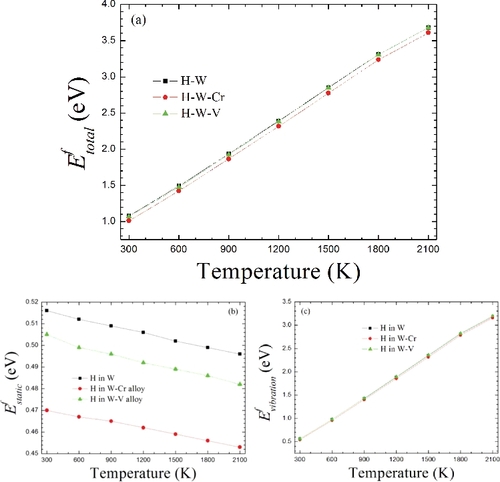
Figure 3. The formation energies of H at the TS with the increase of temperature in W and W-based alloys. (a) Here, the total formation energy only referring to the static H chemical potential μH(T = 0 K). (b) The corresponding static formation energy in reference to the static H chemical potential μH(T = 0 K) based on EquationEquation (15)(15)
(15) and it is related to the lattice expansion. (c) Phonon vibration formation energy. Here, it does not refer to any H chemical potential since it is only the difference between H–W-alloy supercell and pure W-alloy supercell, according to EquationEquation (16)
(16)
(16) .
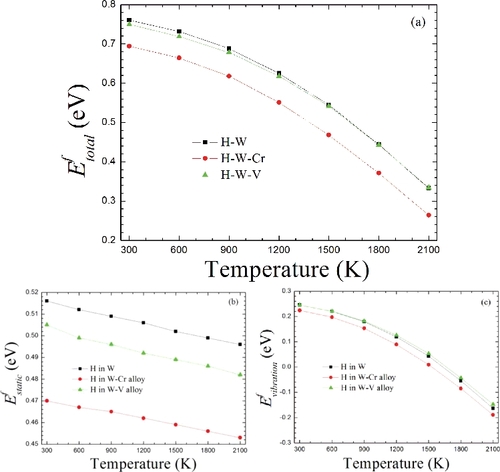
Figure 4. Solubility of H as a function of the reciprocal of temperature at one atmosphere pressure in W and W-based alloys. The experimental solubility of H in W is given from Frauenfelder [Citation63].
![Figure 4. Solubility of H as a function of the reciprocal of temperature at one atmosphere pressure in W and W-based alloys. The experimental solubility of H in W is given from Frauenfelder [Citation63].](/cms/asset/fd126c17-7cb8-484d-ab19-03f8a16ad44b/tnst_a_1383210_f0004_oc.jpg)
Figure 5. (Top view from the [001] direction) The different neighbor (1nn, 2nn and 3nn) t→t and t→o→t diffusion routes around the substitute Cr/V (gray ball) in W (red ball) atomic structure plane supercell. The red and black arrows represent t→t and t→o→t routes, respectively. The TS and OS are denoted by triangle and circle, respectively.
![Figure 5. (Top view from the [001] direction) The different neighbor (1nn, 2nn and 3nn) t→t and t→o→t diffusion routes around the substitute Cr/V (gray ball) in W (red ball) atomic structure plane supercell. The red and black arrows represent t→t and t→o→t routes, respectively. The TS and OS are denoted by triangle and circle, respectively.](/cms/asset/dd8d4902-8406-46c2-bec1-c6769e7a2afc/tnst_a_1383210_f0005_oc.jpg)
Figure 6. Along the t→t diffusion route, the H-migrating energy barriers with the temperature in W and W-based alloys. (a) The total energy barriers. (b) The energy barriers from the lattice expansion contribution. (c) The energy barriers from phonon vibration contribution.
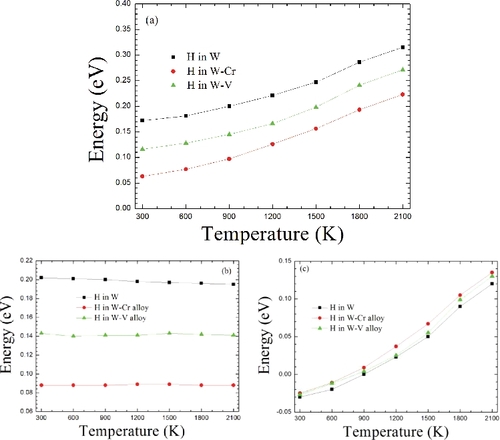
Figure 7. Along the t→o→t diffusion route, the H-migrating energy barriers with the temperature in W and W-based alloys. (a) The total energy barriers. (b) The energy barriers from the lattice expansion contribution. (c) The energy barriers from phonon vibration contribution.
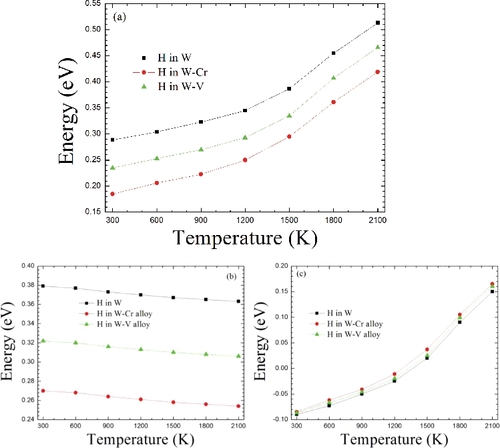
Figure 8. Along the t→t route, the diffusivity (D) of H as a function of reciprocal of temperature in W and W-based alloys.
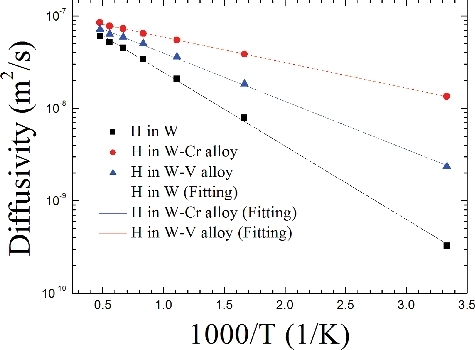