Figures & data
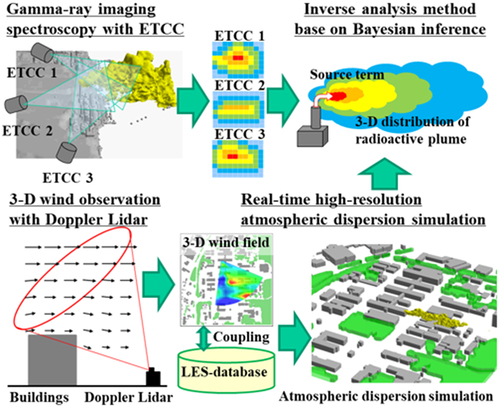
Figure 2. Schematic view of ETCC and schematic explanation of difference in PSF between the conventional Compton camera and ETCC.
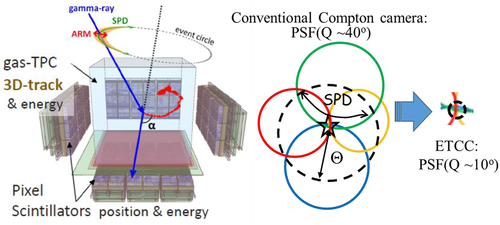
Figure 3. Typical structure of boundary layer flows over buildings and 3D wind field measurement by Doppler lidar.
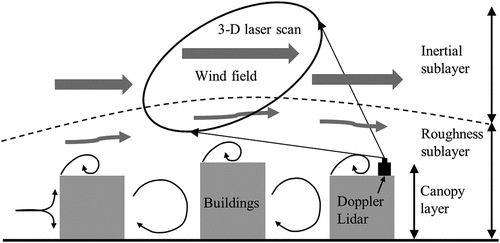
Figure 4. Schematic illustration of a calculation setup to generate the gamma-ray response function matrix.
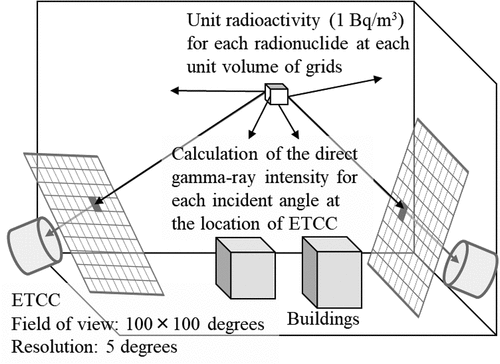
Figure 5. Flow of the inverse analysis method to obtain the temporally changing release rate and 3D distribution of radioactive plume.
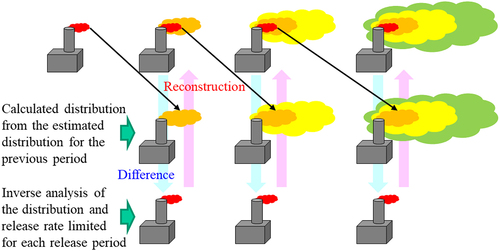
Figure 6. Inverse analysis domain setting. Green marks and arrows at the four corners of the domain indicated the locations and horizontal view angles of ETCCs. Examples of gamma-ray images at ETCC locations are also shown.
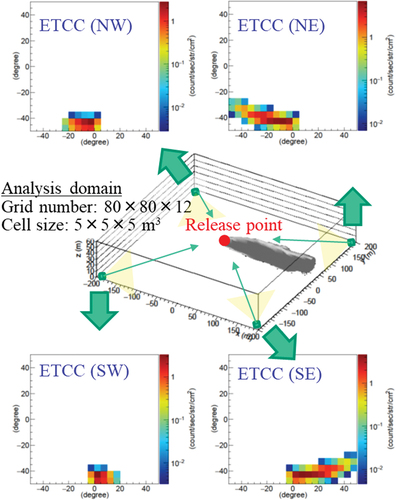
Figure 7. Hypothetical gamma-ray images by ETCCs located at (a) NW, (b) NE, (c) SW, and (d) SE corners of the analysis domain for the 622-keV gamma-ray of 137Cs for the first 1-min period of the test case.
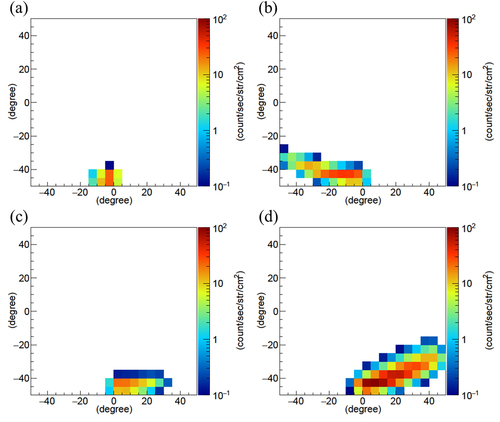
Figure 8. Scatterplots of air concentrations of 137Cs comparing the ground truth with (a) the prior condition normalized by applying the release rate and (b) inverse analysis result of the optimum case for the first 1-min period of the test case. The solid and broken lines indicate the perfect and FAC2 lines, respectively.
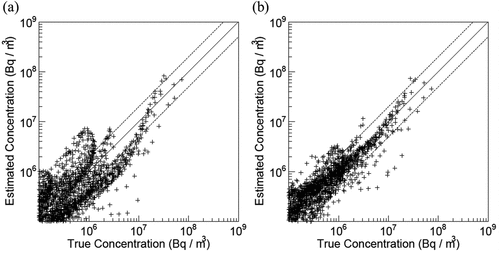
Figure 9. Horizontal distributions of air concentrations of 137Cs at the level of the release height for (a) the ground truth, (b) inverse analysis result of the optimum case, and (c) the prior condition normalized by applying the release rate for the first 1-min period of the test case.
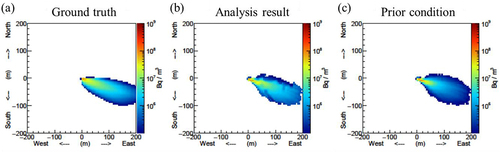
Table 1. Release rate and the maximum air concentration for the ground truth, the prior condition, and the inverse analysis results for 40 cases for the first 1-min period and FAC2 comparing air concentrations between the ground truth and analysis results.
Table 2. Release rate and the maximum air concentration for the second 1-min period by the ground truth and the inverse analysis results for the five cases, in which the release rates for the second 1-min period were 0.1, 0.5, 1.0, 2.0, and 10.0 times the release rate of the first 1-min period, together with FAC2 comparing air concentrations between the ground truth and analysis results.
Figure 10. Scatterplots of air concentrations of 137Cs comparing the ground truth with the inverse analysis results for the cases with (a) 0.1, (b) 0.5, (c) 1.0, (d) 2.0, and (e) 10.0 times the release rate. The solid and broken lines indicate the perfect and FAC2 lines, respectively.
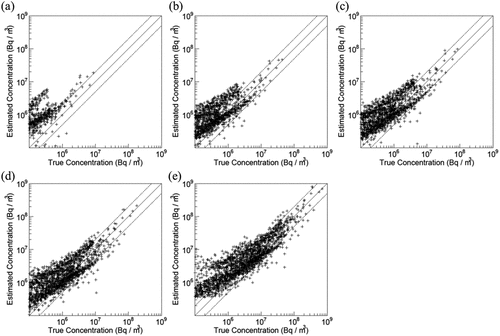
Table 3. Release rate and the maximum air concentration for the second 1-min period by the original and modified inverse analysis results for the five cases, in which the release rates for the second 1-min period were 0.1, 0.5, 1.0, 2.0, and 10.0 times the release rate of the first 1-min period, together with FAC2 comparing air concentrations between the original and modified analysis results.
Table 4. Characteristics of radionuclides, gamma-ray energies, emission rates, and attenuation coefficients in air for 137Cs, 134Cs, 131I, 132I, 133I, 132Te, 133Xe, and 85Kr, and the maximum values of the hypothetical gamma-ray intensities by ETCC for these radionuclides generated using the same air concentration distribution for the first 1-min period.