Figures & data
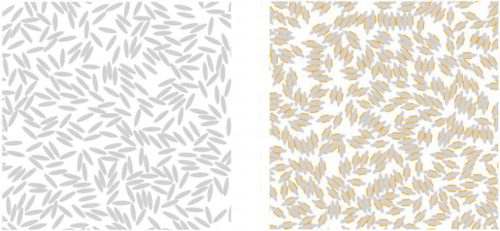
Figure 1. Schematic figure visualising the geometric definition of the patches in our model. For reasons of clarity only two of the up to four patches are shown; they can be positioned at the (co-)vertices of the elliptic body. The impenetrable, elliptic particle is defined via its semi-axes a and b; for this particular representation a value was assumed. The osculating circles of the (co-)vertices with radii
and
are marked by the pink lines. The patches (highlighted in pink) are approximated by sectorial regions of two concentric circles: the inner one is the osculating circle of the respective (co-)vertex while the outer circle has a radius which is by
or
larger than
or
, defining thus the interaction range of the patch. Inside the patch region the interaction is assumed to be constant. The widths of the patches are measured by the opening angles
and
(as seen from the centres of the osculating circles) or
and
(as seen from the centre of the ellipse).
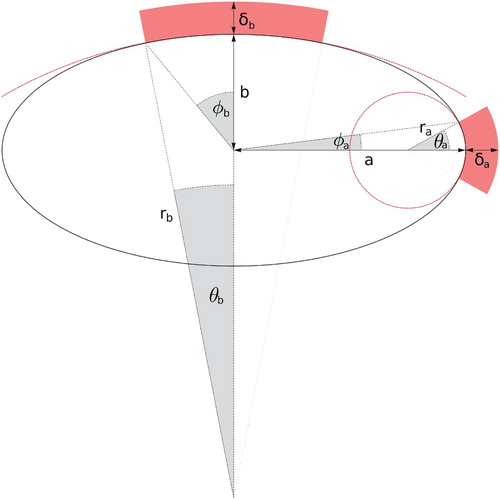
Table 1. Values that specify the maximum extent of patches in our model, as specified by a criterion put forward in the text: 
and 
– maximum opening angles of the patches as seen from the centres of the respective osculating circles, 
and 
– maximum opening angles of the patches as seen from the centre of the ellipse, and 
and 
– maximum patch extents as measured in units of the elliptic circumference.
Figure 2. Schematic representations of elliptic patchy particles as they have been used in this contribution; patches are highlighted in orange. Particles with aspect ratios (panels a and b), and 6 (panel c) have been considered. In all cases the interaction range was chosen to be
(in units of
); the opening angles
(panels a and b) and
(panel c) are chosen such that the criterion for approximating the ellipse by its osculating circle within the patch region is fulfilled with an accuracy of
(see text and Table ).
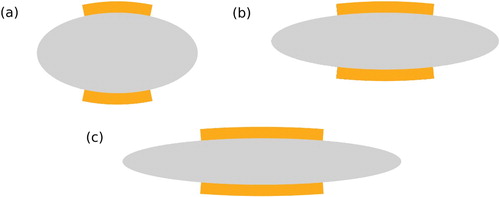
Figure 3. Representative snapshots taken from our NPT MC simulations, showing particles in a section of the simulation cell. Results have been obtained for patchy elliptic particles with aspect ratio and reduced, dimensionless pressure
. Selected temperatures are:
– top left,
– top right,
– bottom left, and
– bottom right.
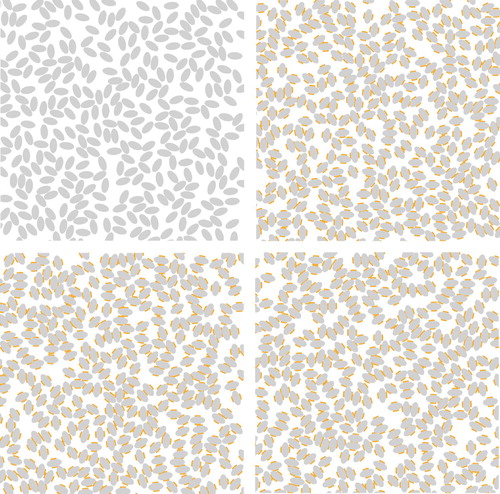
Figure 4. Representative snapshots taken from our NPT MC simulations, showing particles in a section of the simulation cell. Results have been obtained for patchy elliptic particles with aspect ratio and reduced, dimensionless pressure
. Selected temperatures are:
– top left,
– top right,
– bottom left, and
– bottom right.
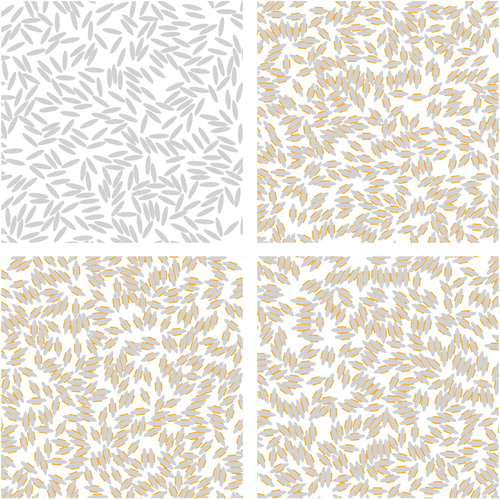
Figure 5. Representative snapshots taken from our NPT MC simulations, showing particles in a section of the simulation cell. Results have been obtained for patchy elliptic particles with aspect ratio and reduced, dimensionless pressure
. Selected temperatures are:
– top left,
– top right,
– bottom left, and
– bottom right.
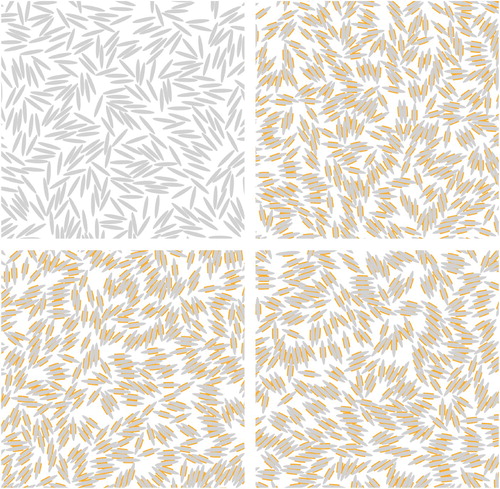
Figure 6. Radial distribution functions as functions of r (in reduced units of
) for κ- and
-values as specified on top of each panel and for different temperatures (as labelled in the panels). The vertical arrows in each of the panels indicate for the respective κ-values the distances r = 2a and r = 2b (again in reduced units
).
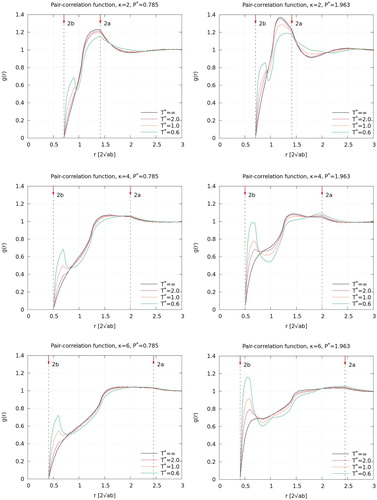
Figure 7. Equation-of-state, i.e. as a function of the packing fraction η for four different temperatures (as labelled), including the case of undecorated, hard ellipses (i.e. for
; in the latter case our results are consistent with the data presented in [Citation9]. Results are shown for three different κ-values: top panel –
, central panel –
, and bottom panel –
. The insets show the trivial low-density equation-of-state of the system.
![Figure 7. Equation-of-state, i.e. P⋆ as a function of the packing fraction η for four different temperatures (as labelled), including the case of undecorated, hard ellipses (i.e. for T⋆=∞; in the latter case our results are consistent with the data presented in [Citation9]. Results are shown for three different κ-values: top panel – κ=2, central panel – κ=4, and bottom panel – κ=6. The insets show the trivial low-density equation-of-state of the system.](/cms/asset/cae4aa20-b7ee-4b63-89a1-f12d0f2471ee/tmph_a_1592254_f0007_oc.jpg)