Figures & data

Figure 1. Level scheme with potential energy curves for H and the three-laser excitation pathway. The first laser, photolysing H
S, produces high-v levels in the electronic ground state; the second laser induces the F−X transitions; the third laser ionises the molecule at energies above 131,000 cm−1. All excitations occur at the large internuclear separation
4–5 a.u, indicated by the grey (yellow) shaded bar. See text for further details.

Figure 2. Experimental configuration. Pulsed dye laser (PDL I) provides UV pulses for photolysis of HS molecules. The nascent H
photoproducts are subjected to two-photon Doppler-free spectroscopy, using 305 nm pulses generated by an injection-seeded narrowband PDA laser. A third laser (PDL II) is employed for recording autoionisation resonances excited from the F outer well levels. Ions are extracted by the electrostatic lens and detected at the end of the time-of-flight (TOF) tube after mass-selection.
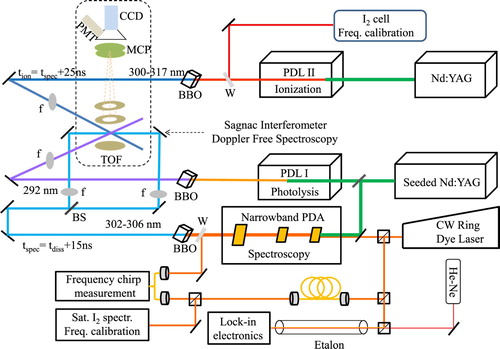
Figure 3. Doppler-free two-photon recording of the line calibrated using etalon markers with an FSR of 300.01 MHz and with respect to a reference
hyperfine line of 127I
at 16,377.60438 cm−1 [Citation42].
![Figure 3. Doppler-free two-photon recording of the Q(7) line calibrated using etalon markers with an FSR of 300.01 MHz and with respect to a reference (B−X) P(94) (12,3) a15 hyperfine line of 127I2 at 16,377.60438 cm−1 [Citation42].](/cms/asset/714bd2b8-a243-402c-a243-a08075077888/tmph_a_1599457_f0003_oc.jpg)
Figure 4. (a) Recorded spectra of the
line under various power densities; (b) ac-Stark extrapolation plot of the
transition for determining the field-free value of the transition frequency.
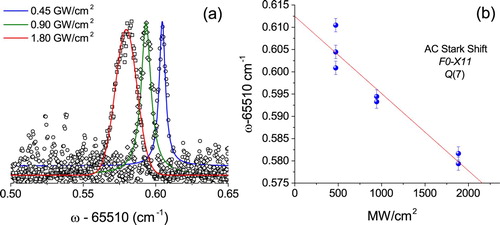
Table 1. Results of high precision measurements on two transitions in the 
band. The experimentally-derived level energies are compared with the results from MQDT calculations for the F state [Citation9]. Values are given in cm−1.
Figure 5. Autoionisation spectra recorded from ,
and
states in para-H
. Common resonances with J=5 calculated from MQDT are indicated.
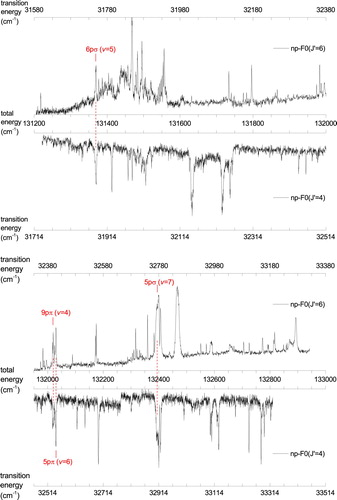
Figure 6. Autoionisation spectra recorded from ,
and
states in ortho-H
. Common resonances with J=6 calculated from MQDT are indicated. The (
) limit of the complex resonance Rydberg series is also shown.

Figure 7. Complex resonance in ortho-H recorded from both
,
and
intermediate states. The vertical lines indicate members of an np Rydberg series converging to a limit with
and
at 131,339.66 cm−1. The red circles represent the calculated level energies, numbered consecutively from n=36, i.e. including interlopers. The approximate weight of the
interloper in the wave function of each level is indicated on the ordinate and provides a rough measure of the intensity transferred from the interloper to the Rydberg series. Another interloper
is also identified in the MQDT analysis as explained in the main text.
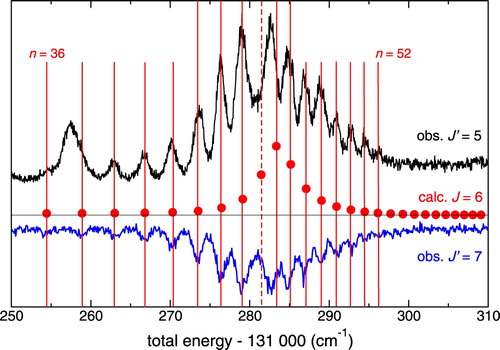
Table 2. Autoionisation resonances for J=5 and J=6 angular momenta observed in the spectra of Figures and , here assigned and compared with calculations via MQDT theory. Values are given in cm−1.
Figure 8. Effective quantum defects derived from the observed peaks in Figure . The MQDT analysis reveals the presence of two interlopers in this complex resonance, see text for details.
