Figures & data
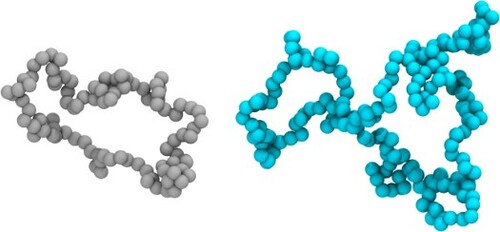
Figure 1. (a) Probability of finding an unknotted configuration in the sample of simulated rings. The black solid line indicates the best fit for
(
). The error bars indicate the standard error over two independent simulation runs. (b) Probability of finding a specific knot configuration in the sample of simulated rings for few N.
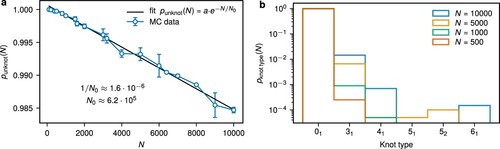
Figure 2. (a) Scaling of the ring's mean square radius of gyration with the polymerisation degree N. The black line is the fit of the MC results (open blue circles), yielding
for
. Right inset: normalised distributions of
for different ring lengths N. Left inset: snapshot of a ring with
. (b) Scaling of the mean area of the ring's minimal surface with N. The black line is the fit
of the simulation results (open blue circles), yielding
for
. Right inset: normalised distributions of
for different ring lengths N. Left inset: snapshot of a minimal surface of a ring with
.
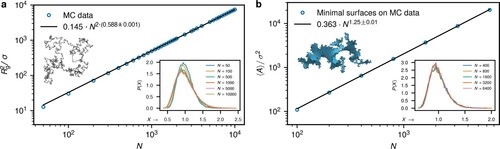
Figure 3. Effective interactions between monodisperse ring polymers at infinite dilution. (a) The effective potential between a pair of rings of the same length N for different N. (b) The steric part of the effective potential
that does not account for the non-concatenation condition. (c) The topological part of the effective potential
. (d) The comparison between
,
, and
for
.
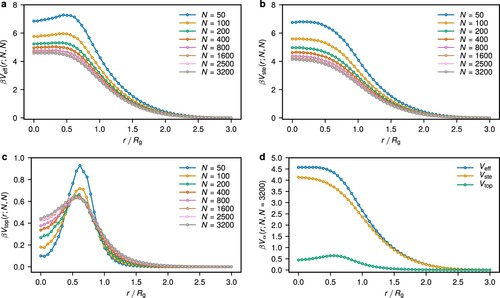
Figure 4. Effective interactions between polydisperse ring polymers at infinite dilution. (a) The effective potential between a pair of rings of length
and
. The same length ratios
are shown with the same color. (b) The steric part of the effective potential
that does not account for the non-concatenation condition. (c) The topological part of the effective potential
. (d) The amplitude of the effective potential,
shown for different
as a function of
. The dashed gray line indicates the apparent scaling
. The inset highlights the fact that the behaviour of the zero-separation value of the effective potential as a function of
is bracketed between the asymptotic behaviours
and
, see the text.
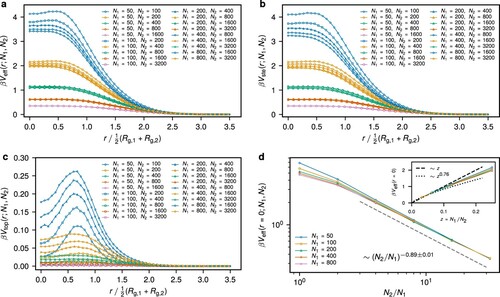
Figure 5. The ratio between the topological and effective potential as a function of the centre-of-mass separation between two rings r for different length ratios
.
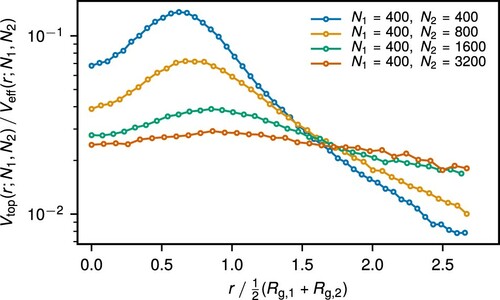
Figure 6. Threading properties as a function of ring centre-of-mass separation r and the length ratio . Probability of finding a threading conformation as a function of r for (a) monodisperse and (b) polydisperse ring pairs.
is the probability that the ring i threads the ring j placed at a given centre-of-mass distance r.
, which quantifies the depth of threading, computed on the threading ring pairs (
, solid lines) and all ring pairs (dashed lines) as a function of r for (c) monodisperse and (d) polydisperse ring pairs. (e) Distribution of
(including cases with
) at different fixed distances between the rings' centres of mass for the case with
and
. Distribution of (f)
and (g)
(including cases with
) at different fixed distances between the rings' centres of mass for the case with
and
.
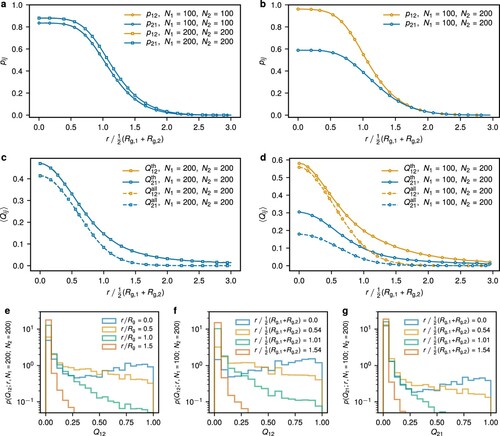