Figures & data

Figure 1. Vertical transition energies computed by the DLPNO-STEOM method for a set of symmetrically substituted indigo dyes. Data plotted from .
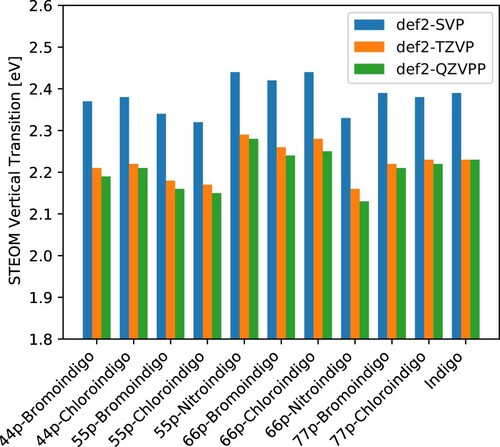
Table 1. Total energies for the and
states of the H chromophore
. The excitation energy to the S1 state, ω, is computed in eV. Here, we understand ‘STEOM’ to mean ‘DLPNO-STEOM’ with identical settings as in the remaining calculations in this paper. All other methods are canonical.
Table 2. Vertical transition energies computed by the DLPNO-STEOM method for a set of symmetrically substituted indigo dyes.
Table 3. Solvation effects compared to gas-phase STEOM calculations. Solvents sorted with increasing dielectric constant ε from left to right.
Table 4. Benchmark results: the CPCM-corrected DLPNO-STEOM excitation energy (ω), the VG correction , the 0–0 transition energy (
), the experimental absorption band maxima (
) [Citation13] and the error of the total STEOM estimate with respect to experiment (
).
Table 5. Statistical evaluation of the VG corrections () and the errors in DLPNO-STEOM 0–0 transition energies (
) from Table with respect to the experimental values (eV).
Figure 2. Normalized error density distribution with respect to experiment, , from Table , with a Gaussian probability density function overlaid.
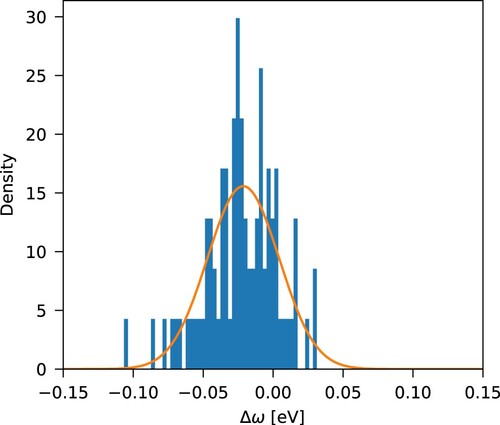
Figure 3. The effect of chemical substitutions on excitation energies. The dashed line indicates perfect agreement between theory and experiment, the shaded zone signifies the region within which the agreement is within ±0.1 eV.
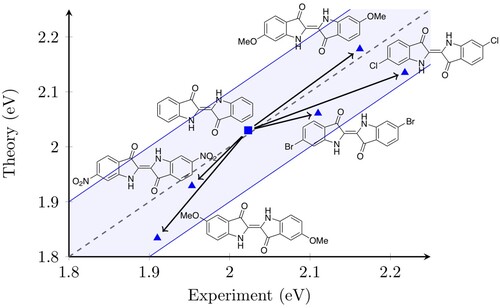
Figure 4. Normalized VIS absorption spectra for indigo, 6-bromoindigo, and 6,6'-dibromoindigo in DMSO. The unshifted computed spectra are presented with an inhomogeneous line width of as well as sticks for each vibrational mode. The experimental spectra were obtained from Ref. [Citation83].
![Figure 4. Normalized VIS absorption spectra for indigo, 6-bromoindigo, and 6,6'-dibromoindigo in DMSO. The unshifted computed spectra are presented with an inhomogeneous line width of 700cm−1 as well as sticks for each vibrational mode. The experimental spectra were obtained from Ref. [Citation83].](/cms/asset/3a74f1b2-305d-4409-b4ea-c1acacdef0f6/tmph_a_1965235_f0004_oc.jpg)
Figure 5. The spectrum of Tyrian purple and the 0–0 transition energies of three of its components studied in this paper. The shaded area corresponds to the region where indirubin dyes absorb (its width chosen from the spread between the band maxima of indigorubin dyes in Ref. [Citation83] with an additional in both directions).
![Figure 5. The spectrum of Tyrian purple and the 0–0 transition energies of three of its components studied in this paper. The shaded area corresponds to the region where indirubin dyes absorb (its width chosen from the spread between the band maxima of indigorubin dyes in Ref. [Citation83] with an additional 500cm−1 in both directions).](/cms/asset/b5d6e461-0dad-49cd-89f7-39eeff87aed4/tmph_a_1965235_f0005_oc.jpg)