Figures & data
Figure 2. Potential energy curves for equilateral geometries of calculated using UHF, spin-purified UHF (sp-UHF), and CCSD. (a) The
,
and
states in the absence of a magnetic field. (b) The corresponding
,
and
states in the presence of a magnetic field of magnitude
. For states with
, the potential energy curves for the lowest energy orientation with the field vector parallel to the y-axis in Figure is shown. For the state with
, the lowest energy orientation with the field vector parallel to the x-axis (perpendicular to the molecular plane) is shown. (c) and (d) The corresponding
,
and
states in the presence of a magnetic field of magnitude 0.5 B0 and 1.0
, respectively.
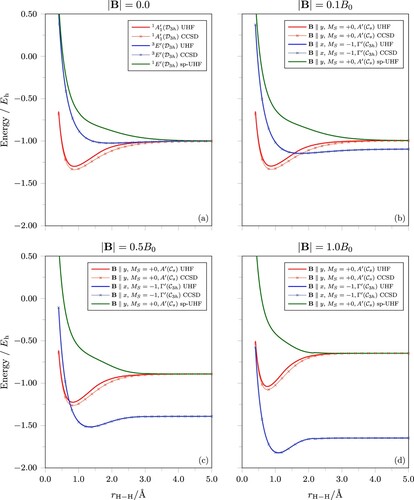
Figure 3. Potential energy curves of the lowest state for equilateral geometries of H3 at various magnetic field strengths. In all finite-field cases, the field vector is parallel to the x-axis and perpendicular to the molecular plane. At zero field, the system has
unitary symmetry in which the state is given the symmetry label
. At finite fields, the system is reduced to
unitary symmetry and the state becomes
with spin-projection degeneracies lifted. (a) The UHF and CCSD energy curves. (b) The corresponding UHF and CCSD interaction energy curves given by
plotted on a smaller vertical scale to show the variation of the minimum structures of the UHF and CCSD energy curves in (a) with respect to magnetic field strengths more clearly.
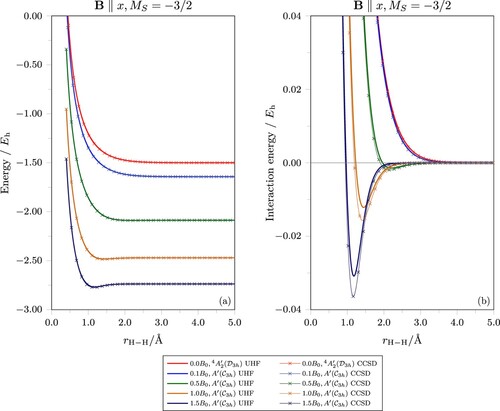
Table 1. Optimised molecular structures for the and H3 molecules in particular electronic states as a magnetic field is applied either along the x (
) or y (
) directions as shown in Figure .
Figure 4. Variation of the lowest UHF solution of
in the presence of a uniform magnetic field
across a range of
-constrained geometry-optimised structures. For each constrained value of
, all three H−H bond lengths in
are allowed to relax to attain an optimal geometry. (a) Energies of the two occupied
MOs in
along this path, plotted relative to the energy of the HOMO of the
geometry-optimised structure in each field strength. The forms of the two β MOs at
,
, and
are also shown: the isosurface for MO
is plotted at
, and the colour at each point
on the isosurface indicates the phase angle
at that point according to the accompanied colour wheel. (b) Energy of the
UHF solution along this path, plotted relative to the value at the
geometry-optimised structure in each field strength.
![Figure 4. Variation of the lowest MS=−1 UHF solution of H3+ in the presence of a uniform magnetic field B∥x across a range of θ213-constrained geometry-optimised structures. For each constrained value of θ213, all three H−H bond lengths in H3+ are allowed to relax to attain an optimal geometry. (a) Energies of the two occupied ms=−1/2 MOs in H3+ along this path, plotted relative to the energy of the HOMO of the θ213=180∘ geometry-optimised structure in each field strength. The forms of the two β MOs at 60∘, 120∘, and 180∘ are also shown: the isosurface for MO φi(r) is plotted at |φi(r)|=0.08, and the colour at each point r on the isosurface indicates the phase angle argφi(r)∈(−π,π] at that point according to the accompanied colour wheel. (b) Energy of the MS=−1 UHF solution along this path, plotted relative to the value at the θ213=180∘ geometry-optimised structure in each field strength.](/cms/asset/9456993e-e1ba-4725-8451-23233988b1b4/tmph_a_2152748_f0004_oc.jpg)
Figure 5. Isosurfaces of occupied MOs in the lowest UHF solutions in
and H2 in the presence of a perpendicular magnetic field. For each molecule, the optimal geometry at
is used to calculate the UHF solution, which is then tracked smoothly to
with the help of the MOM as the field is switched off while keeping the geometry fixed. This is to generate the corresponding MOs at zero field for investigation purposes. The isosurface for MO
is plotted at
, and the colour at each point
on the isosurface indicates the phase angle
at that point according to the colour wheel shown in Figure .
These MOs turn out to be slightly symmetry-broken at zero field, both of which having actual symmetry
. This symmetry breaking, however, is not discernible from the isosurface plots and thus does not affect the qualitative argument given in the main text. Furthermore, as the perpendicular field is introduced, the MOs become symmetry-conserved.
![Figure 5. Isosurfaces of occupied MOs in the lowest MS=−1 UHF solutions in H3+ and H2 in the presence of a perpendicular magnetic field. For each molecule, the optimal geometry at |B⊥|=1.0B0 is used to calculate the UHF solution, which is then tracked smoothly to B=0 with the help of the MOM as the field is switched off while keeping the geometry fixed. This is to generate the corresponding MOs at zero field for investigation purposes. The isosurface for MO φi(r) is plotted at |φi(r)|=0.08, and the colour at each point r on the isosurface indicates the phase angle argφi(r)∈(−π,π] at that point according to the colour wheel shown in Figure 4.†,‡ These MOs turn out to be slightly symmetry-broken at zero field, both of which having actual symmetry A1′⊕E′. This symmetry breaking, however, is not discernible from the isosurface plots and thus does not affect the qualitative argument given in the main text. Furthermore, as the perpendicular field is introduced, the MOs become symmetry-conserved.](/cms/asset/ae795386-fba8-4a10-b014-49c39a8cfa2f/tmph_a_2152748_f0005_oc.jpg)
Figure 6. Variation of the lowest UHF solution of H3 in the presence of a uniform magnetic field
across a range of
-constrained geometry-optimised structures. For each constrained value of
, all three H−H bond lengths in H3 are allowed to relax to attain an optimal geometry. (a) Energy of the
UHF solution along this path, plotted relative to the value at the
geometry-optimised structure in each field strength. (b)–(e) Energies of the three occupied β MOs plotted relative to the energy of the HOMO of the
geometry-optimised structure in each field strength. The forms of the β MOs at
,
, and
are also shown: the isosurface for MO
is plotted at
, and the colour at each point
on the isosurface indicates the phase angle
at that point according to the colour wheel shown in Figure .
![Figure 6. Variation of the lowest MS=−3/2 UHF solution of H3 in the presence of a uniform magnetic field B∥x across a range of θ213-constrained geometry-optimised structures. For each constrained value of θ213, all three H−H bond lengths in H3 are allowed to relax to attain an optimal geometry. (a) Energy of the MS=−3/2 UHF solution along this path, plotted relative to the value at the θ213=180∘ geometry-optimised structure in each field strength. (b)–(e) Energies of the three occupied β MOs plotted relative to the energy of the HOMO of the θ213=180∘ geometry-optimised structure in each field strength. The forms of the β MOs at 60∘, 120∘, and 180∘ are also shown: the isosurface for MO φi(r) is plotted at |φi(r)|=0.08, and the colour at each point r on the isosurface indicates the phase angle argφi(r)∈(−π,π] at that point according to the colour wheel shown in Figure 4.](/cms/asset/6ea6c8bb-9dd0-4e7e-a5a0-1194110ab240/tmph_a_2152748_f0006_oc.jpg)