Figures & data
Figure 2. The electronic spectrum of C (top) and H
O@C
(bottom), recorded under the same laboratory conditions. The data are photofragmentation spectra of C
−He and H
O@C
−He complexes.
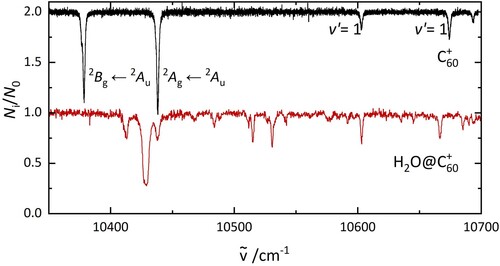
Table 1. Vibrational and translational modes obtained from calculations.
Figure 3. Number of HO@C
−He complexes (N
) as a function of laser fluence upon irradiation at 10,438 cm
. Without exposure to laser radiation, 1100±60 ions with m/z = 742 were in the trap. 800±30 complexes remain after irradiation, corresponding to 73±5% of the population.
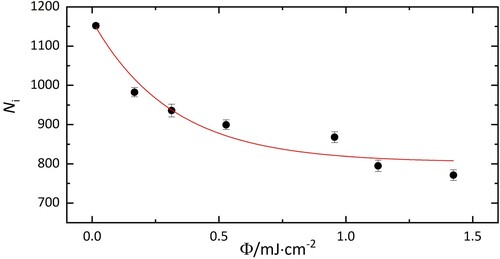
Figure 4. Electronic spectra of HO@C
(top), p-H
O@C
(middle), and o-H
O@C
(bottom). The lower two traces were obtained in 2 colour experiments. The middle spectrum was recorded by fragmenting all complexes that absorb at 10,429 cm
. The bottom spectrum was recorded after irradiation at 10,438 cm
.
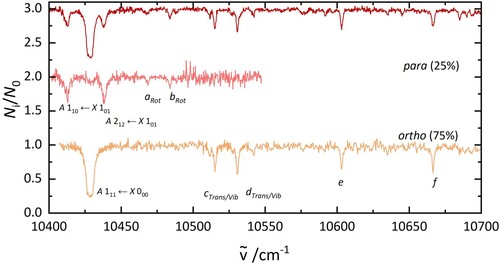
Figure 5. PGopher simulations of the rotational pattern of an electronic transition of HO. The details of the simulations are explained in the text. The middle trace uses the parameters based on a geometry optimisation (B3LYP/6-31++G**) while the upper one shows a fit involving a geometry change of H
O between ground and excited state.
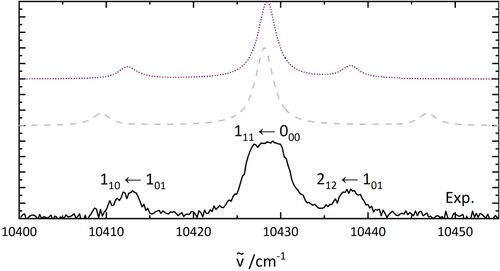
Table 2. Rotational constants used for the simulation in Figure .
Table 3. Absorption energies and proposed assignment of bands in the HO@C
and D
O@C
spectra.
Figure 6. A comparison of the electronic spectrum of HO@C
(top) and D
O@C
(bottom) recorded under similar laboratory conditions. The labels are discussed in the text.
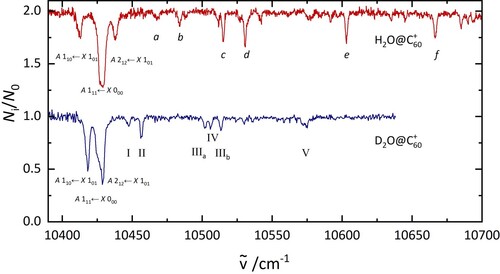