Figures & data
Figure 1. The Waikakahi Stream catchment (South Canterbury, New Zealand) showing the locations of the eight surveyed 1 km stream segments. The stream flows from west to east before it joins the Waitaki River c. 4 km upstream of the coast. All tributaries and the mainstem 1 km upstream of Site 8 are ephemeral.
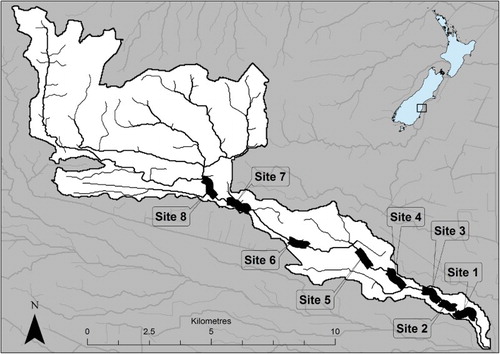
Table 1. Mean percentage values (and standard deviations) for instream habitat variables, including mesohabitat type, depths and sediment cover, for all 20 m sub-reaches combined (n = 115). Stream bed sediment categories were only for the visible areas of the stream bed (i.e. areas not obscured by deep water or aquatic macrophytes).
Table 2. Mean percentages (and standard deviations) for the coverage of various riparian vegetation categories for all GDZ (n = 86).
Table 3. Correlations from an exploratory regression analysis of all riparian and instream habitat variables.
Figure 2. Regression between longitudinally weighted mean % fine sediment cover and mean fenced riparian area per 100 m of stream length (n = 23) for all GDZ within each stream segment (1000 m). Circle size represents the weight (0 through 10) applied to each % fine sediment response value according to the location of the 100 m reach within the 1 km survey segment. For example, fine sediment cover values from a reach at the top of a survey segments were weighed one 10th the value of a reaches at the bottom of the segment.
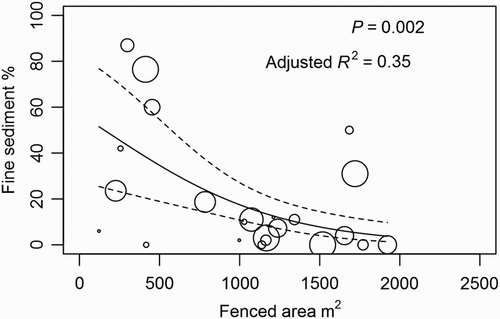
Figure 3. Summary of P, adjusted R2 and sample size values for 10 individual (unweighted) sequential regressions of instream fine sediment cover vs mean fenced riparian area. The first regression, at the 100 m reach scale, compares sediment cover in instream survey reaches to fenced riparian area from adjacent GDZ. For each subsequent regression, the fenced riparian area is the mean from upstream GDZ incrementally included in the analysis.
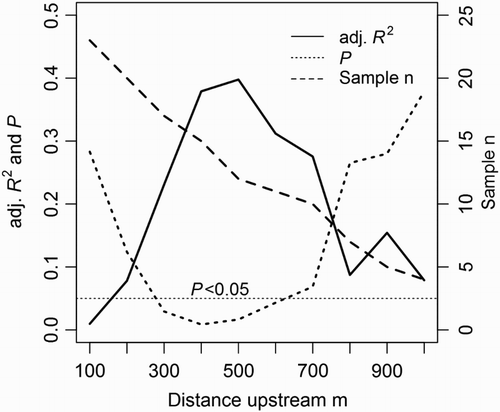
Figure 4. Mean deposited fine sediment cover from 100 m instream reaches regressed against the mean fenced riparian area of four upstream continuous 100 m GDZ (n = 15). The relationship shown (the fourth regression in a sequence of 10 regressions that included predictor variables from upstream areas in 100 m increments) had the strongest R2 value.
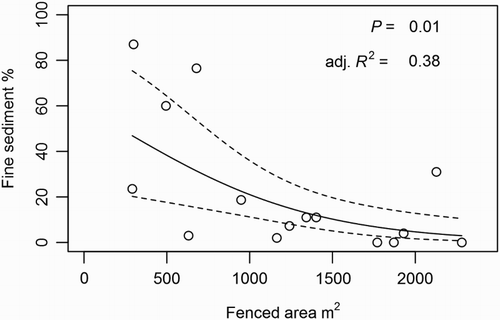
Figure 5. A, Linear regression between mean density (no./m2) of longfin and shortfin eels (combined) and wetted width (of instream survey reaches) in November 2012; B, mean trout biomass (g/m2) and overhanging (draping) vegetation expressed as mean % cover of the wetted width in November 2012; C, total trout biomass (g) and wetted width in March 2013.
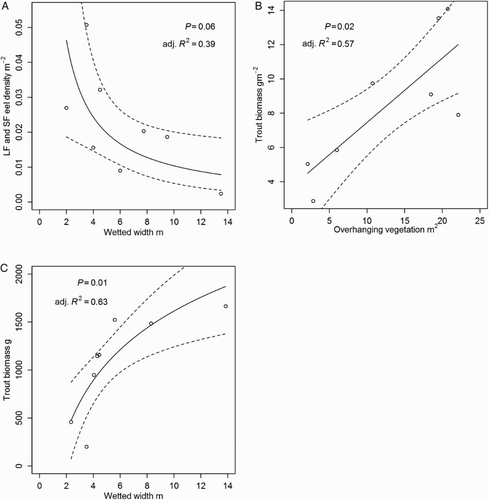