Figures & data
TABLE I Main Properties from the Design Specification for the MSFR
Fig. 1. Layout of the MSFR, from Ref. [Citation16].
![Fig. 1. Layout of the MSFR, from Ref. [Citation16].](/cms/asset/21739d2b-e577-4277-9021-81f84eb9c636/unse_a_2250144_f0001_oc.jpg)
TABLE II Employed Energy Groups and Relative Coefficients for Albedo Boundary Conditions
TABLE III Fission Yields at for the Investigated Isotopes
TABLE IV Decay Constants for Radioactive GFPs
Fig. 5. Initial conditions for quantities of interest: (a) pressure, (b) temperature, (c) velocity, and (d) fission rate with the 2D geometry.
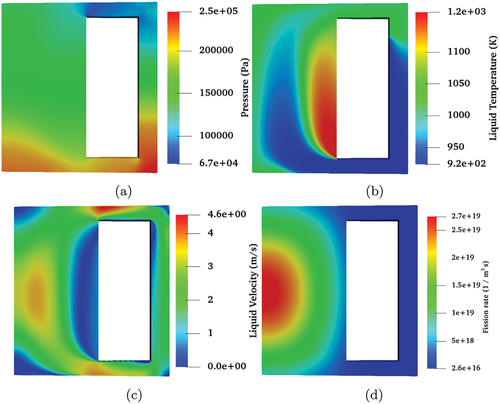
Fig. 6. Initial conditions for quantities of interest: (a) pressure, (b) temperature, (c) velocity, and (d) fission rate with the 3D geometry.
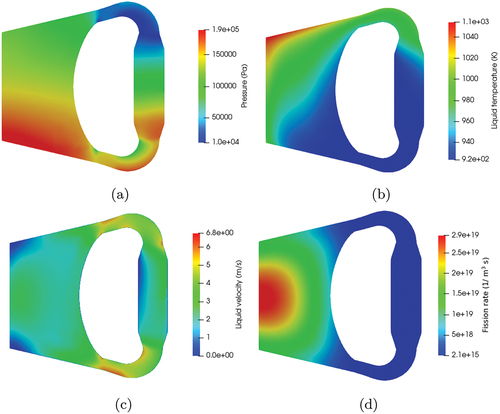
TABLE V Initial Inventories for the Various GFPs in 2D Simulations
TABLE VI Initial Inventories for the Various GFPs in 3D Simulations
Fig. 8. Efficiency in 2D geometry: (a) time evolution of the cycle time at various helium flow rates and (b) illustration of the inverse proportionality between the cycle time
and the helium flow rate. The coefficient of determination R2 is 0.994 at 20 s and 0.992 at 30 s.
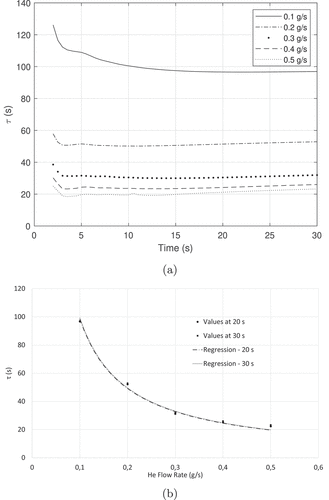
Fig. 9. Efficiency in 3D geometry: (a) time evolution of the cycle time at various helium flow rates and (b) illustration of the inverse proportionality between the cycle time
and the helium flow rate. The coefficient of determination R2 is 0.988.
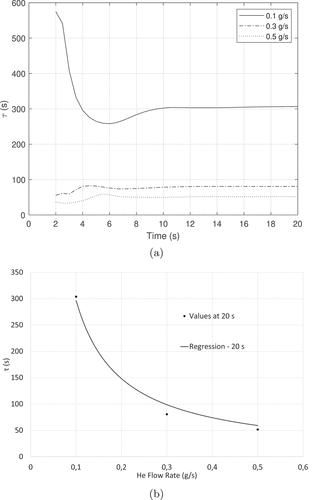
TABLE VII Comparison Among Cycle Times Found in Two Dimensions and Three Dimensions*
TABLE VIII Comparison Among Cycle Times Found in Two Dimensions and Three Dimensions Against the Average Helium Fraction*
TABLE IX Contributions to Activity and Decay Heat of the Removed Gas Found with the 3D Geometry*
Fig. 11. Activity leaving the core in the gas per unit time found with the 3D geometry, referred to the whole core. Notice the logarithmic scale.
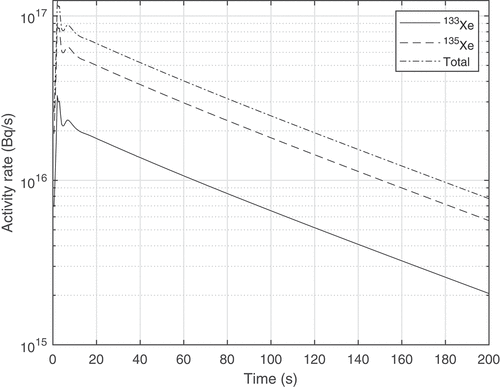
TABLE X Void Coefficient at Various Helium Flow Rates in 2D Geometry
Fig. 16. Volume fraction of the gas at the beginning (left) and after 10s (right) of loss of helium removal. Notice the common scale.
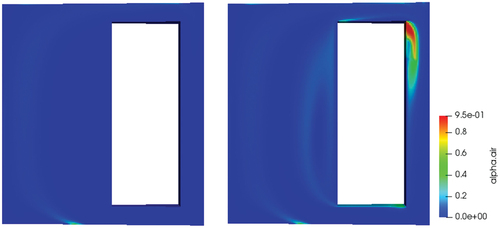
Data Availability Statement
The data that support the findings of this study are openly available in Zenodo at http://doi.org/10.5281/zenodo.7515490.