Figures & data
Figure 1. Tyre schematic for the pure longitudinal problem (). The bristles and the tyre carcass (modelled as a linear spring) are drawn in red. The generalised forces acting on the tyre-wheel system are represented in blue. The speeds are finally given in green. The dot notation stands for total derivative with respect to time. The local variable
is referred as the distance from the leading edge.
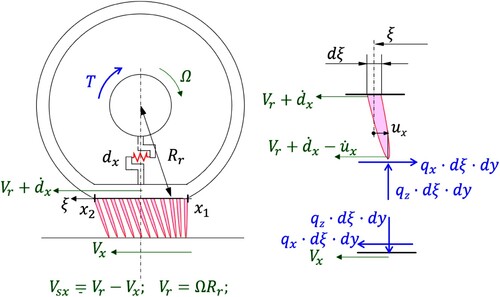
Figure 2. Time trend for the shear stresses in the contact patch for case I. The three figures refer to different values of the nondimensional travelled distance
. Since the trend for the solution
in the adhesion region is constant, the steady-state condition is reached when
, or, equivalently, when the travelled distance equals the contact length.
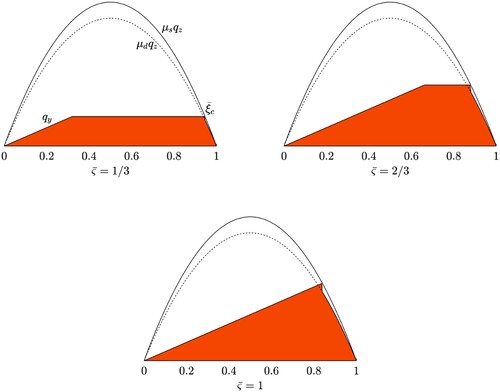
Figure 3. Time trend for the shear stresses in the contact patch for case II. The three figures refer to different values of the nondimensional travelled distance
. Since the trend for the solution
in the adhesion region is constant, the steady-state condition is reached when
, or, equivalently, when the travelled distance equals the value
.
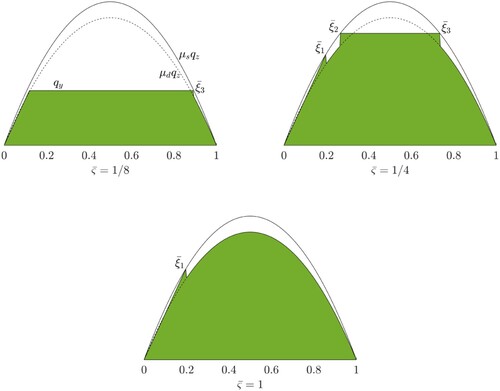
Figure 4. Time trend for the shear stresses in the contact patch for case III. The three figures refer to different values of the nondimensional travelled distance
. Since the trend for the solution
in the adhesion region is constant, the steady-state condition is reached when
, or, equivalently, when the travelled distance equals the value
.
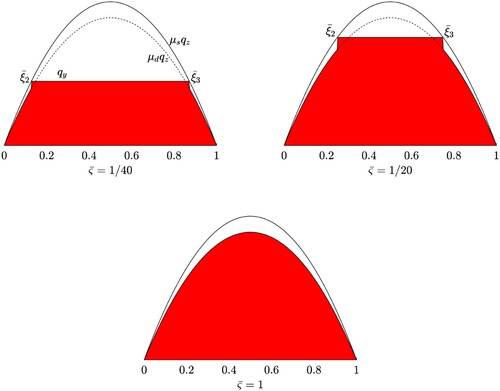
Figure 5. Time trend for the shear stresses in the contact patch due to pure camber. The three figures refer to different values of the nondimensional travelled distance
. Since the trends for the solutions
and
in the adhesion region are parabolic and linear, respectively, the steady-state condition is only reached when
= 1, or, equivalently, when the travelled distance equals the contact length.
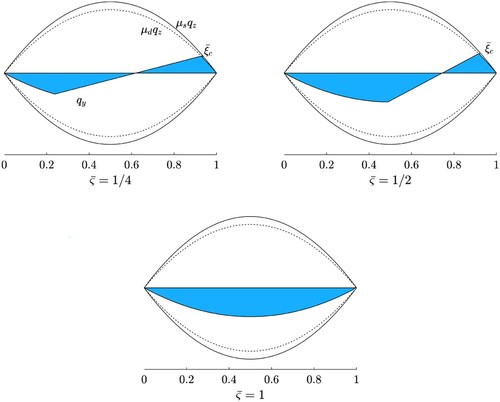
Figure 6. Time trend for the shear stresses in the contact patch due to pure lateral interaction and pure camber, respectively, starting from nonzero initial conditions
. The figures refer to different values of the nondimensional travelled distance
. The initial condition for the pure lateral case (left-hand side panel) corresponds to a transient trend for the deformation of the bristles due to an initial slip value
. The transient solution for the camber (right-hand side panel) refers to a case in which the initial spin value
has the same sign as the current one. The initial condition corresponds to a steady-state trend of the bristle displacement.
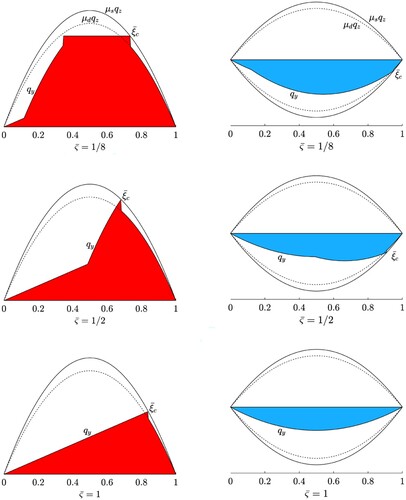
Table