Figures & data
Figure 1. Heating from a single bubble exposed to 1 MHz HIFU at 2.8 MPa pressure amplitude. Power deposited via (a) viscous boundary layer heating and (b) absorption of secondary acoustic emissions (SAE) are plotted as a function of the equilibrium bubble radius and the viscosity of the medium Citation[99], Citation[139]. The linear resonance radius at 1 MHz is 3.72 µm.
![Figure 1. Heating from a single bubble exposed to 1 MHz HIFU at 2.8 MPa pressure amplitude. Power deposited via (a) viscous boundary layer heating and (b) absorption of secondary acoustic emissions (SAE) are plotted as a function of the equilibrium bubble radius and the viscosity of the medium Citation[99], Citation[139]. The linear resonance radius at 1 MHz is 3.72 µm.](/cms/asset/08926c36-ce8a-43d3-8839-24cc1eabbb2e/ihyt_a_219335_f0001_b.gif)
Figure 2. Schematic of the HIFU generation (dashed line), passive cavitation detection (continuous line) and B-mode imaging (dotted line) apparatus.
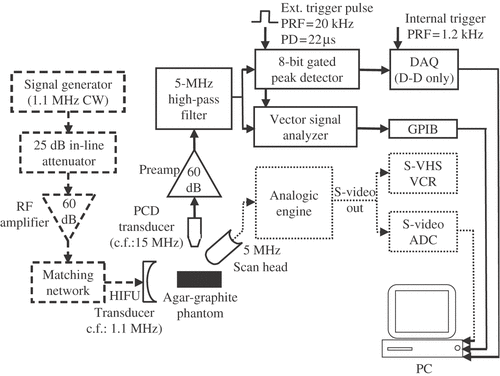
Figure 3. (a) Measured temperature rise (labeled ‘Themocouple) and PCD output (labeled ‘PCD') as a function of time for a 1-s 1.1-MHz HIFU insonation of an agar-graphite tissue phantom at three different pressure amplitudes. No inertial cavitation occurs in (iii), whilst cavitation onsets halfway through the exposure in (ii) and at the start of exposure in (i). In (ii) and (iii), there is a dramatic increase in the observed rate of heating that is coincident with the onset of inertial cavitation activity. (b) Peak temperature rise with respect to ambient conditions vs. peak-positive acoustic pressure for the agar/graphite.
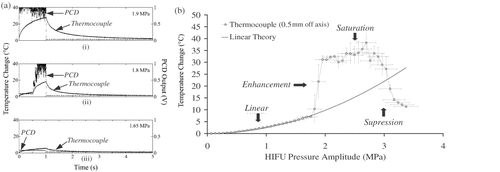
Figure 4. Comparison between peak PCD output voltage, the frequency spectra of the PCD-received traces and the pre- and post-HIFU B-mode images obtained whilst ramping up the input voltage to the HIFU transducer every 5 s (bottom x-axis), thus increasing the peak negative focal pressure in steps of 0.15 MPa (top x-axis). The confocal position of the HIFU, PCD and imaging transducers in the agar-graphite phantom is indicated by the white cross on the B-mode images, with the HFU transducer being to the left of the image, the PCD transducer pointing out of the image and the imaging transducer lying above the image. At a peak negative focal pressure of 0.9 MPa, a sudden increase in peak PCD voltage is observed, which is coincident with a clear jump in broadband noise emissions. However, a hyperechogenic region only becomes visible on the B-mode image at t = 2 s once the HIFU excitation amplitude had been ramped up to 1.35 MPa. This strongly suggests that the appearance of a bright-up on B-mode images cannot be assumed to be coincident with the onset of inertial cavitation activity.
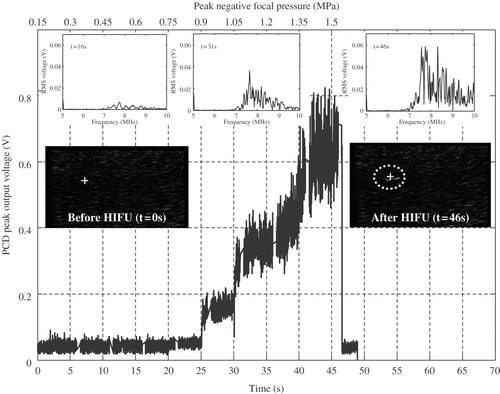
Figure 5. RMS voltage received by a passive cavitation detector (PCD) positioned confocally with the HIFU transducer during excitation of an agar-graphite tissue phantom at a 2.89 MPa peak negative focal pressure amplitude. In all cases, the phantom was exposed to 1-s continuous-wave (CW) excitation, followed by a further 60 s either CW exposure (dotted line) or 20% duty-cycle excitation (continuous dark grey, light grey and black lines). A rapid decay in cavitation activity is observed during sustained CW exposure, suggesting movement of the bubble cloud towards the HIFU transducer and out of the field of view of the PCD. Different implementations of 20% duty cycle HIFU excitations, using either 5 cycles (dark grey), 10 cycles (light grey) or 100 cycles (black continuous) on-time, make it possible to sustain cavitation activity in the focal region for extended periods of time. The 10-cycle implementation appears most effective at sustaining the initially generated level of inertial cavitation activity.
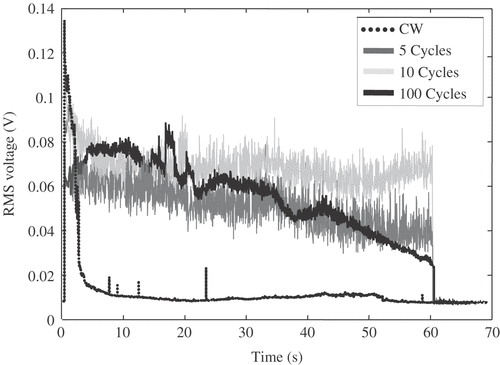