Figures & data
Figure 1. Sketch showing the superposition of a series of heat-up and cool-down processes for a particular point in the heated target. (a) Ideally, heat from the previous settings should almost completely dissipate before the next setting is applied. Images acquired at the time points shown by circles can be subtracted to directly obtain the temperature for each heat setting (denoted by line X-Y for the second heat setting). (b) In reality, due to time limitations, the effect of the previous settings is exponentially decayed. The image corresponding to the second heat setting is denoted by line X-Y.
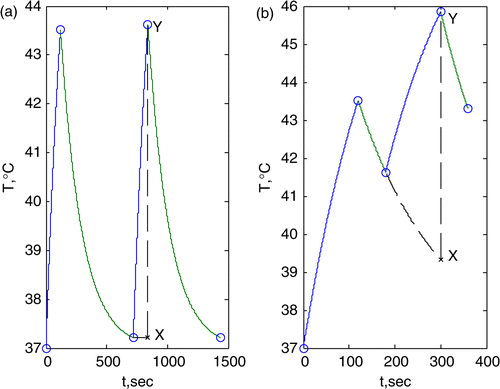
Figure 2. Patient upper leg finite element model, shown within the 140 MHz mini-annular phased array heating apparatus (red for tumor, blue for bone, green for muscle and yellow for fat).
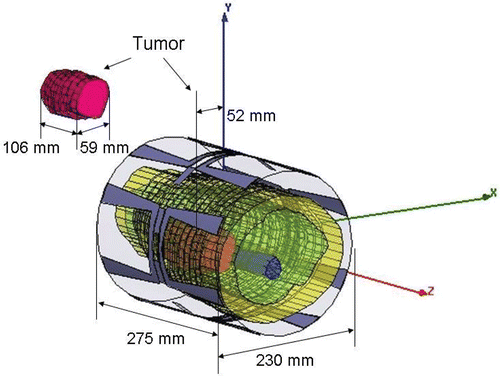
Table I. Nominal properties’ values (assumed for 140 MHz).
Table II. Property deviations.
Table III. Uncertainty in antenna phase and magnitude settings.
Figure 3. Temperature distribution at a central axial slice through tumor (z = 0 cm) at the end of the feedback iteration number indicated in top right of each panel. The first driving vector was the maximum eigenvector from an initial model with property deviations. The outermost ring is fat, within which is the muscle region. The larger irregular object on the left side of the muscle region is tumor, and the smaller irregular object at the center is bone. The colored regions are: red for ≥43°C, yellow for ≥ 41°C, light blue for ≥40°C and blue for ≥39°C.
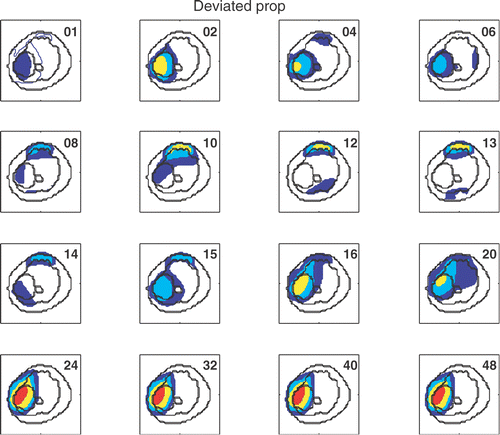
Figure 4. Temperature distribution at a central axial slice through tumor (z = 0 cm) at the end of the feedback iteration number indicated in top right of each panel. The first driving vector was the maximum eigenvector of the idealized model shifted by 2 cm. Geometry and labels are the same as .
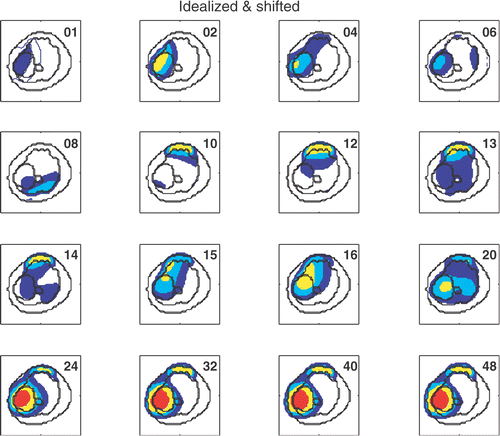
Figure 5. (a) Temperature distribution at different axial locations along the thigh at the 49th iteration, for an initial driving vector corresponding to the maximum eigenvector of the erroneous models described at the top of each panel. Geometry and labels are the same as . (b) Temperature distributions at different z-locations using the 49th driving vector when the first heating vector was chosen as the minimum eigenvector for the four numerical validations. Geometry and labels are the same as in . For patient position shift, unlike , focusing is not centered within the tumor target.
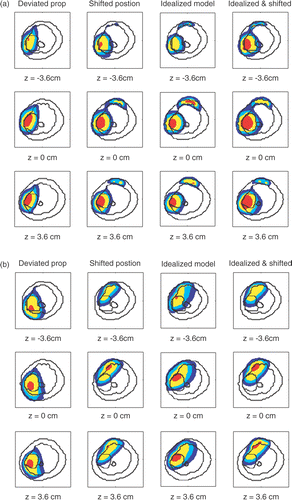
Figure 6. (a) The percentage of heated tumor volume (T ≥ 43°C) and normal tissues (T ≥ 41°C), when initial driving vector is the maximum eigenvector of the five initial models. (b) For patient position shift, using the minimum eigenvector as the first heating vector only raises ∼60% tumor temperature above 43°C. (b) The percentage of heated tumor volume (T ≥ 43°C) and normal tissues (T ≥ 41°C), when initial driving vector is the minimum eigenvector of the five initial models. For patient position shift, using the minimum eigenvector as the first heating vector only raises ∼60% tumor temperature above 43°C.
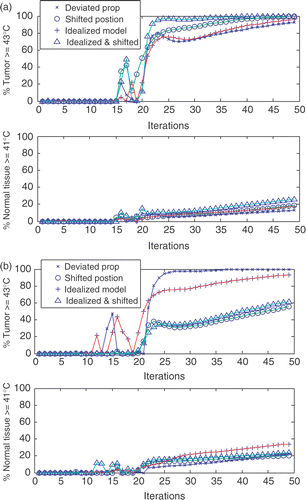
Figure 7. (a) The dot product between the heating vectors at each feedback iteration step to the maximum eigenvector of the standard model, when the first heating vector was obtained from maximal eigenvector for the four numerical validations. (b) The dot product between the heating vectors at each feedback iteration step to the maximum eigenvector of the standard model, when the first heating vector was obtained from the minimum eigenvector for the four numerical validations.
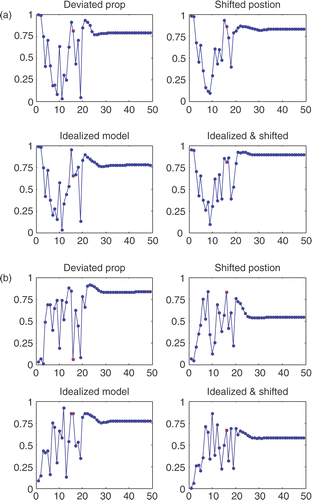
Table IV. List of absolute value of the dot product after convergence.
Figure 8. (a) The percentage of heated tumor volume (T ≥ 43°C) and normal tissues (T ≥ 41°C), when initial driving vector is the maximum eigenvector from an initial model with property deviations in combination with four sets of antenna discrepancies (). In general, more normal tissues are detrimentally heated in the presence of antenna discrepancy. (b) The percentage of heated tumor volume (T ≥ 43°C) and normal tissues (T ≥ 41°C), when initial driving vector is the minimum eigenvector from an initial model with property deviations in combination with four sets of antenna discrepancies (). In general, more normal tissues are detrimentally heated in the presence of antenna discrepancy.
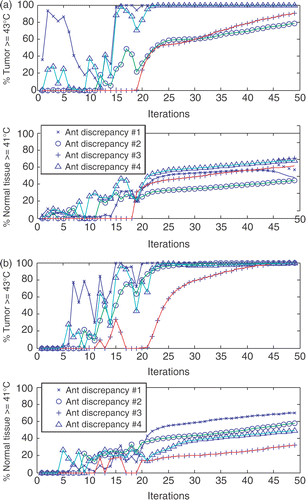