Figures & data
Figure 2. Overview of IRE pulses, with VP [V] as the electric potential (the voltage) of the pulses, tP [s] as the duration of a single pulse, nP as the pulse number, NP as the total number of pulses, τP [s] as the duration between two pulses, and fP [Hz] as the pulse frequency.
![Figure 2. Overview of IRE pulses, with VP [V] as the electric potential (the voltage) of the pulses, tP [s] as the duration of a single pulse, nP as the pulse number, NP as the total number of pulses, τP [s] as the duration between two pulses, and fP [Hz] as the pulse frequency.](/cms/asset/ad0c5e05-3165-4a33-a10d-3005f3bb0503/ihyt_a_1753828_f0002_b.jpg)
Figure 3. Examples of applied boundary conditions for the calculations of the electric potential distribution with arrow representing electric-current flow through the outer surface of the model (IBOS [A]). The red and the blue circles represent BEM with the electrodes given in the color gray, and black lines represent the BOS of the model. (A) shows Dirichlet boundary conditions at BEM and BOS, and (B) shows Dirichlet boundary conditions at BEM, and Neumann boundary conditions at BOS if IBOS = 0 A or Robin boundary conditions if IBOS is limited.
![Figure 3. Examples of applied boundary conditions for the calculations of the electric potential distribution with arrow representing electric-current flow through the outer surface of the model (IBOS [A]). The red and the blue circles represent BEM with the electrodes given in the color gray, and black lines represent the BOS of the model. (A) shows Dirichlet boundary conditions at BEM and BOS, and (B) shows Dirichlet boundary conditions at BEM, and Neumann boundary conditions at BOS if IBOS = 0 A or Robin boundary conditions if IBOS is limited.](/cms/asset/0e9123fa-0b6f-4273-8a88-b05a09e8f77b/ihyt_a_1753828_f0003_c.jpg)
Figure 4. Examples of applied boundary conditions for the calculations of the temperature distribution. The brown circles represent BEM with the electrodes given in the color gray, and black lines represent the BOS of the model. Arrows represent heat flux vector that is normal to the BEM (qBEM [W⋅m−2]) and the BOS (qBOS [W⋅m−2]). At both BEM and BOS (A) shows Dirichlet boundary conditions, and (B) shows Neumann boundary conditions if qBEM = 0 W⋅m−2 and qBOS = 0 W⋅m−2, or Robin boundary conditions if qBEM and qBOS are limited.
![Figure 4. Examples of applied boundary conditions for the calculations of the temperature distribution. The brown circles represent BEM with the electrodes given in the color gray, and black lines represent the BOS of the model. Arrows represent heat flux vector that is normal to the BEM (qBEM [W⋅m−2]) and the BOS (qBOS [W⋅m−2]). At both BEM and BOS (A) shows Dirichlet boundary conditions, and (B) shows Neumann boundary conditions if qBEM = 0 W⋅m−2 and qBOS = 0 W⋅m−2, or Robin boundary conditions if qBEM and qBOS are limited.](/cms/asset/218529c9-fb65-48a0-8312-0942253ab2dd/ihyt_a_1753828_f0004_c.jpg)
Figure 5. (A) Assumed model that includes infinite electrode plates to calculate the temperature increase during IRE. The electrodes are presented as gray rectangles with infinite length and IRE-TR as a yellow rectangle. The black triangle represents a possible location in the model with maximum temperature increase. (B) An example of a temperature increase profile based on EquationEquation 7(7)
(7) , which is divided in normothermia (from tinit to tMH), moderate hyperthermia (MH; from tMH to tTA), and thermal ablation (TA; from tTA to tend).

Figure 6. Examples of electrode configurations applied in studies to calculate the electric-field distribution, with the colors gray and brown as metallic and insulation parts, respectively. These configurations represent: (A) spherical electrodes, (B) needle and surface electrodes, (C) circular plate electrode enclosed by a ring electrode, (D, E) plate electrodes, (F) endovascular electrodes; contralateral electrodes have the same electric potential, (G) needle electrodes, (H) bipolar needle electrode; contralateral electrodes have different electric potential. In each configuration, the distances d [m] and D [m] were defined for the calculation of the energy density. Several studies assumed the needle and bipolar electrodes to be cylinders in 3D models. Other studies assumed the needle and spherical electrodes to be circles in 2D models.
![Figure 6. Examples of electrode configurations applied in studies to calculate the electric-field distribution, with the colors gray and brown as metallic and insulation parts, respectively. These configurations represent: (A) spherical electrodes, (B) needle and surface electrodes, (C) circular plate electrode enclosed by a ring electrode, (D, E) plate electrodes, (F) endovascular electrodes; contralateral electrodes have the same electric potential, (G) needle electrodes, (H) bipolar needle electrode; contralateral electrodes have different electric potential. In each configuration, the distances d [m] and D [m] were defined for the calculation of the energy density. Several studies assumed the needle and bipolar electrodes to be cylinders in 3D models. Other studies assumed the needle and spherical electrodes to be circles in 2D models.](/cms/asset/518fbf93-7562-4b66-b3a7-f13e88e656ff/ihyt_a_1753828_f0006_c.jpg)
Table 1. Summary of organ-dependent electrical and thermal characteristics for cancerous and healthy tissues (see Supplementary Appendix 6 – Table A6.2).
Figure 8. (A) The study characteristics ‘Tissue type’, ‘ΔTmax’, ‘σinit’, ‘Electrode type’, and ‘u’ were plotted as function of ‘Pulse voltage (VP [V])’ and ‘Total pulsing time (tP ⋅ NP [s])’ in logarithmic scale. For a proper clarification of the data points, an extended version can be found in Supplementary Appendix 4 Figure A4.1. The range of ΔTmax was 0.27 ≤ ΔTmax [°C] ≤ 135, which was represented by the linearly scaled red-yellow color bar with the range 0 ≤ ΔTmax [°C] ≤ 40. The ΔTmax values that exceeded 40 °C were 50 °C at (750 V, 9 × 10−3 s) [Citation34], 55 °C at (1500 V, 1 × 10−2 s) [Citation64], 85 °C at (2000 V, 3 × 10−4 s) [Citation55], and 135 °C at (2000 V, 3 × 10−4 s) [Citation55]. Furthermore, the range of u was 1.19 × 105 ≤ u [J⋅m−3] ≤ 11.42 × 108, which was represented by the linearly scaled blue-magenta color bar with the range 0 ≤ u [J⋅m−3] ≤ 10 × 108. The u values that exceeded 10 × 108 J⋅m−3 were two times the value 11.42 × 108 J⋅m−3 at (2000 V, 9 × 10−3 s) [Citation44]. (B) Here, we only plotted the data that was obtained from the pulse parameters which were applied in the experiments. The range of ΔTmax was 1 ≤ ΔTmax [°C] ≤ 39, and the range of the energy density was 2.92 × 106 ≤ u [J⋅m−3] ≤ 8.22 × 108. (C) is similar to (B), with the exception of having ‘Total treatment time (NP ⋅ fP−1 [s])’ in logarithmic scale instead of ‘Total pulsing time’. Please note the change in Y-axis in (C) in comparison to (B), and that the electrical conductivity is the conductivity before the treatment.
![Figure 8. (A) The study characteristics ‘Tissue type’, ‘ΔTmax’, ‘σinit’, ‘Electrode type’, and ‘u’ were plotted as function of ‘Pulse voltage (VP [V])’ and ‘Total pulsing time (tP ⋅ NP [s])’ in logarithmic scale. For a proper clarification of the data points, an extended version can be found in Supplementary Appendix 4 Figure A4.1. The range of ΔTmax was 0.27 ≤ ΔTmax [°C] ≤ 135, which was represented by the linearly scaled red-yellow color bar with the range 0 ≤ ΔTmax [°C] ≤ 40. The ΔTmax values that exceeded 40 °C were 50 °C at (750 V, 9 × 10−3 s) [Citation34], 55 °C at (1500 V, 1 × 10−2 s) [Citation64], 85 °C at (2000 V, 3 × 10−4 s) [Citation55], and 135 °C at (2000 V, 3 × 10−4 s) [Citation55]. Furthermore, the range of u was 1.19 × 105 ≤ u [J⋅m−3] ≤ 11.42 × 108, which was represented by the linearly scaled blue-magenta color bar with the range 0 ≤ u [J⋅m−3] ≤ 10 × 108. The u values that exceeded 10 × 108 J⋅m−3 were two times the value 11.42 × 108 J⋅m−3 at (2000 V, 9 × 10−3 s) [Citation44]. (B) Here, we only plotted the data that was obtained from the pulse parameters which were applied in the experiments. The range of ΔTmax was 1 ≤ ΔTmax [°C] ≤ 39, and the range of the energy density was 2.92 × 106 ≤ u [J⋅m−3] ≤ 8.22 × 108. (C) is similar to (B), with the exception of having ‘Total treatment time (NP ⋅ fP−1 [s])’ in logarithmic scale instead of ‘Total pulsing time’. Please note the change in Y-axis in (C) in comparison to (B), and that the electrical conductivity is the conductivity before the treatment.](/cms/asset/fa8aff05-0f47-4dc4-807c-c1c4dea4ed8c/ihyt_a_1753828_f0008_c.jpg)
Figure 9. Linear regression plots of R3ΔT13 and RΔT13 data points as function of SE-IRE(th). For a proper clarification of the data points, an extended version can be found in Supplementary Appendix 4 Figure A4.2. The ΔTmax and the u ranges were 1.9 ≤ ΔTmax [°C] ≤ 39, and 0.29×107 ≤ u [J⋅m−3] ≤ 8.44 × 107. Please note that the scale of the color bar of the energy density in this figure is different than the scales in . In addition, the symbols at (4.83 × 10−5 m2, 0.32%) and (4.83 × 10−5 m2, 9.19%) have a gray background since the ΔTmax was not reported.
![Figure 9. Linear regression plots of R3ΔT13 and RΔT13 data points as function of SE-IRE(th). For a proper clarification of the data points, an extended version can be found in Supplementary Appendix 4 Figure A4.2. The ΔTmax and the u ranges were 1.9 ≤ ΔTmax [°C] ≤ 39, and 0.29×107 ≤ u [J⋅m−3] ≤ 8.44 × 107. Please note that the scale of the color bar of the energy density in this figure is different than the scales in Figure 8. In addition, the symbols at (4.83 × 10−5 m2, 0.32%) and (4.83 × 10−5 m2, 9.19%) have a gray background since the ΔTmax was not reported.](/cms/asset/7316d037-4e36-4687-917c-63930ef8f77e/ihyt_a_1753828_f0009_c.jpg)
Figure 10. (A) Linear regression plot of ΔTmax data points as function of the energy density. (B) Linear regression plots of R3ΔT13 and RΔT13 data points as function of the energy density. Please note the change in X-axis in (B) in comparison to (A).

Figure 11. The estimated time durations for both mild hyperthermia and thermal ablation as function ΔTmax. The term ‘Extracted’ means that ΔtMH and ΔtTA values were directly provided by the included studies, while the term ‘Calculated’ signifies that ΔtMH and ΔtTA were calculated using the assumptions stated in the Subsection 3.2.2.3. Estimation of time durations of mild-hyperthermic and thermally ablative temperatures. Please note that Δt represents the duration of mild-hyperthermic effect (3 °C ≤ ΔTmax < 13 °C) and thermal ablative effect (ΔTmax ≥ 13 °C) of an included study. The ΔTmax only represents the maximal temperature increase obtained from the included study. Also, note that the value of ΔtMH was 0 s for ΔTmax < 3 °C and the value of ΔtTA was 0 s for ΔTmax < 13 °C.
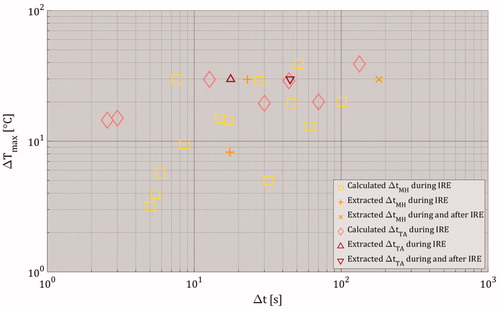