Figures & data
Figure 1. Overview over all 10 RF applicator designs investigated in this work. In the center column, cross-sectional view of the RF arrays depicts the arrangement of the building blocks around the head and details about their position with respect to each other. The rightmost column shows the designs and their positioning for the small tumor model.
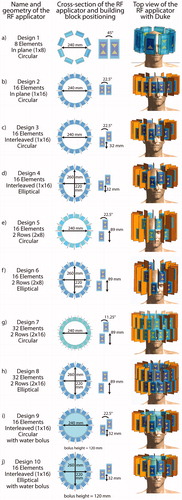
Table 1. Materials and dielectric parameters used for electromagnetic field simulations.
Figure 2. (a) Schematic presentation of the workflow from the diagnostic MRI of the patient to the SAR10g distribution as the hyperthermia treatment planning. (b) Details of the simulation and optimization process from the tumor models to the metrics to be evaluated to assess the RF array for hyperthermia treatment planning and magnetic resonance imaging performance.
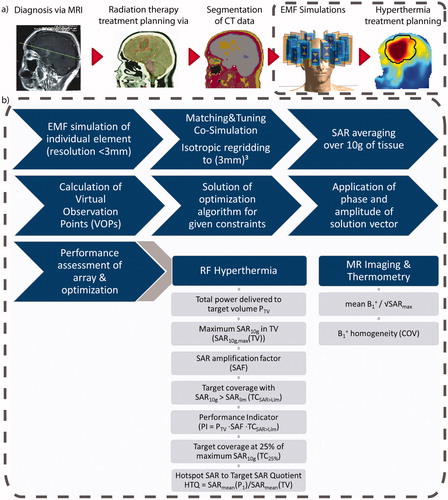
Table 2. Summary of all metrics assessing the performance of the RF-applicators, including the worst reflection and coupling coefficients (column 1, ‘Scattering’), the Hyperthermia Treatment Planning (HTP) results using the power optimization (column 2, ‘Power optimization’), the HTP results using the uniformity optimization (column 3, ‘Uniformity Optimization’) with # indicating the number of additive excitation phase and amplitude settings as well as the results of the B1+ shimming (column 4, ‘MRI’) for the small tumor model (top half) and the large tumor model (bottom half).
Figure 3. Top row: sagittal, coronal and axial section through the small tumor model. Left: description and front view of each design simulated together with the small tumor model; maximum intensity projection of the SAR10g distribution after hyperthermia treatment planning for the power optimization (center) and the uniformity optimization (right).
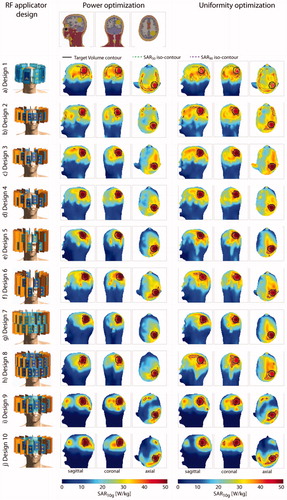
Figure 4. Qualitative comparison of the metrics used to assess HTP quality. The top row displays the results obtained for the small tumor model; the bottom row shows the results for the large tumor model. The results in the left column are obtained using the power optimization; the results of the uniformity optimization are in the right column. Blue lines highlight designs 9 and 10 equipped with a water bolus. All values are normalized to their respective intra-design, intra-optimizer maximum. Metrics labeled with a green font combine to the performance indicator. For better visualization so that higher values are always better, we plotted the reciprocal of the HTQ (1/HTQ = THQ). For the small tumor model, adding the water bolus clearly improved the SAF and pushed toward the highest values for SARmax(TV) and VSAR>Lim. For the large tumor model, the results are very heterogeneous; the water bolus does not add a clear improvement.
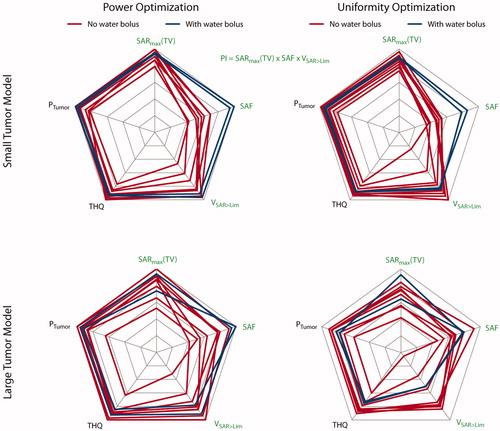
Figure 5. Top row: sagittal, coronal and axial section through the large tumor model. Left: description and front view of each design simulated together with the large tumor model; maximum intensity projection of the SAR10g distribution after hyperthermia treatment planning for the power optimization (center) and the uniformity optimization (right).
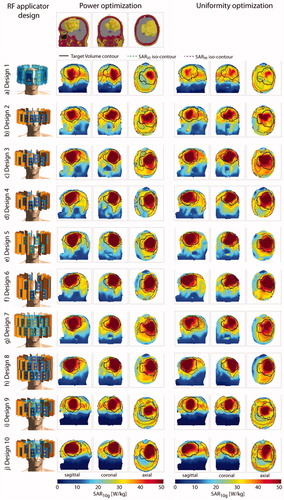