Figures & data
Figure 2. Illustration of the HIFU exposures (orange crosses) on a site and definition of the frame of reference with respect to the anatomy.
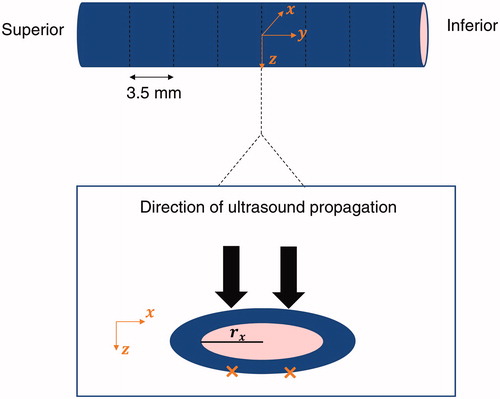
Figure 3. Flowchart of the methodology followed to model the vein wall shrinkage. The ultrasound propagation is first modeled using the k-wave library. From the acoustic simulations, the temperature evolution in tissues is dynamically estimated during the treatment. Based on the updated temperature field, the thermal damage to the vein is computed and the geometry of the vein is updated.
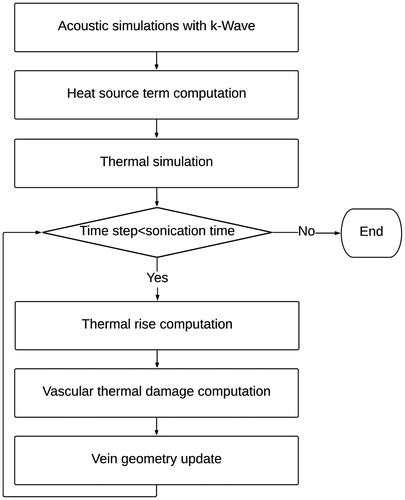
Table 1. Acoustic properties of tissues used for simulations.
Figure 5. Illustration of thermal damage and vein wall constriction process. The vein wall is represented here by concentric rings of blue pixels, having each a unitary length. Based on the updated temperature field modeled by the Pennes bioheat equation, the thermal damage within each voxel is updated and the vein wall constriction (
) is computed. The weighed curvilinear abscissa is then calculated and updated. Based on the special mean of the contracted pixel length, the new venous circumference is computed and the new geometry of the vein is updated. Finally, the thermal damage within each pixel of the updated vein wall is computed.
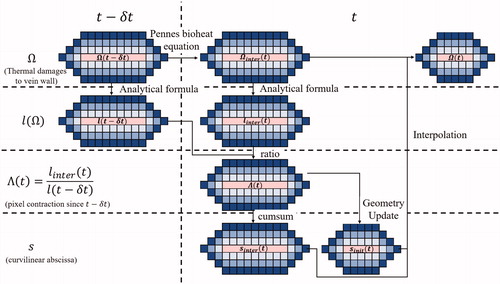
Table 2. Vein diameter reduction measurements.
Figure 7. Ultrasound images of a sheep vein before (a) and immediately after HIFU treatment (b) showing luminal shrinkage and blurred vascular contours.
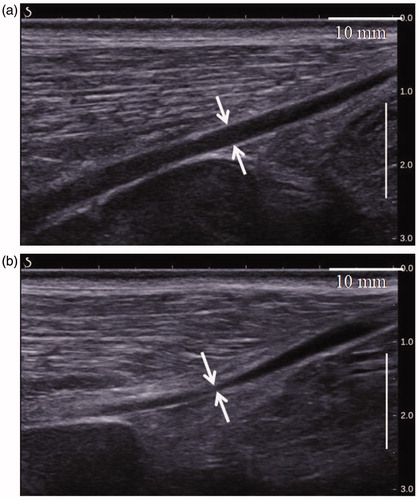
Figure 8. Simulated rms pressure field of the geometrical model in the plane. Pressure is expressed in MPa. Yellow rectangles represent the limits of the subsequent layers.
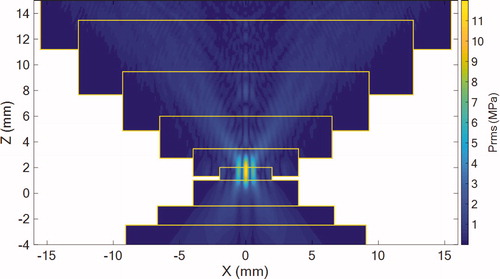
Figure 9. Shrinkage and thermal damage simulations for veins with an initial pretreatment diameter of 2.3 mm (top row), 3.5 mm (middle row) and 5.2 mm (bottom raw) after exposures of the vein. Left column: before treatment. Left column: after treatment. Note that the number of “yellow” pixels does not reflect the percentage of vein wall which is damaged since these pixels correspond to shrunken part of the vein wall. Typically, for the 5.2 mm vein, the coagulated pixels correspond to about 50 % of the initial vein wall.
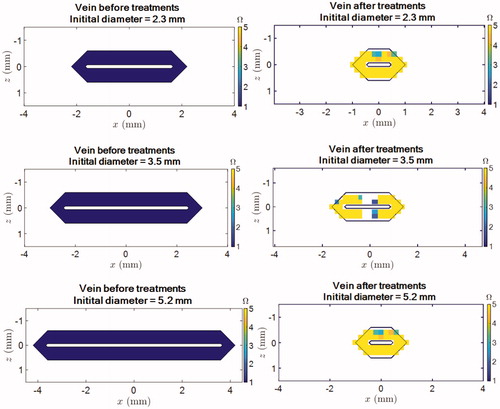
Figure 10. Comparison between the simulated (blue) and experimental (red) luminal shrinkage in one vein cross-section. The error bars refer to the experimental standard deviations () and means are represented by the red circles.
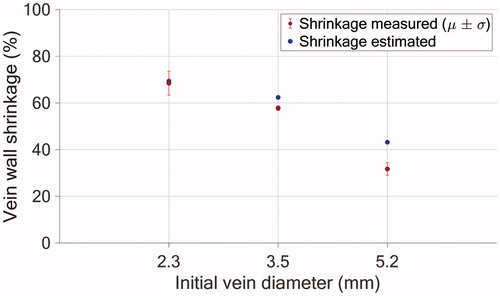
Table 3. Relative errors for the three diameters studied.