Figures & data
FIG. 1 (a) Top view schematic of the optical configuration of the polar nephelometer that shows the placement of the detectors and their apertures. (b) The particles are confined by a sheath flow to the center of the detection array. An electromechanical device rotates the 1/2 wave plate 45° periodically to change the polarization plane of the incident light from parallel to the scattering plane to perpendicular to it.
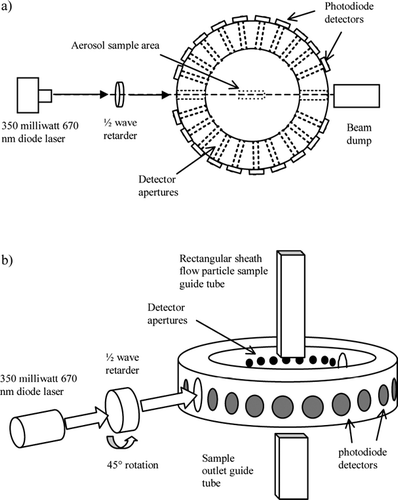
FIG. 2 (a) Geometric view from above the scattering plane that illustrates the parameters of Equation (Equation1), in which only two detector locations are shown for clarity and each detectors field of view (FOV) is shown as a dotted line. Particles are confined by the sample port to intersect the laser beam between –D L to D L . The detector field of view is larger than the length of the laser beam in which particles are present as the detector scattering angle approaches the forward and reverse angles. (b) Diamonds (⧫) are the theoretical expectations for PSL particles with a diameter of 806 nm (line) adjusted to instrument sensing geometry described by Equation (Equation1) with incident light polarized parallel to the measurement plane.
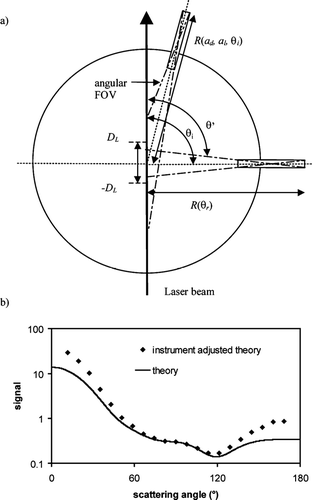
FIG. 3 (a) The angular scattering intensities of PSL spheres with a specified diameter of 360 nm and standard deviation of 5.40 nm measured by the polar nephelometer with incident light polarized parallel (▴) and perpendicular (◊) to the measurement plane with expectations developed via GA search of Mie-Lorenz theory calculations and fitted to the measurement. (b) Similar results but for PSL particles with a specified diameter of 596 nm and a standard deviation of 7.75 nm. (c) Similar results but for PSL particles with a specified diameter of 806 nm and a standard deviation of 11.28 nm.
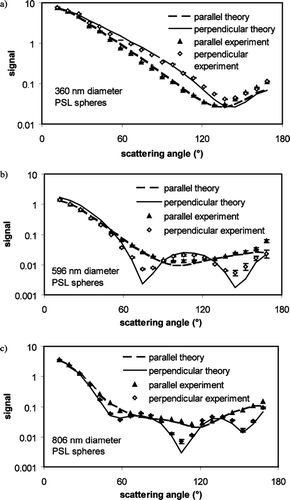
FIG. 4 GA retrieved real refractive index for synthetic experimental results based on PSL spheres with an average particle radius of 180 nm and a standard deviation of 0.054. A random noise level based on the maximum SNR is applied to each channel to produce the error bars that are the standard deviation of 10 separate GA retrievals of the simulated noisy results. The dotted line is the correct mr, which is 1.5854.
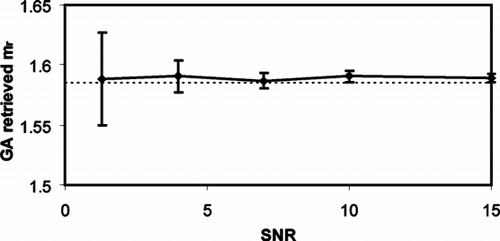
FIG. 5 (a) The change in the GA retrieved mr as a function of the percent error in the measured mean of the particle size distribution. Three different standard deviations are used corresponding to %CV of 1%, 10%, and 30%. These results are based on the retrieval of synthetic experimental results determined using Mie-Lorenz calculations based on PSL spheres. (b) Same as above but for the multi-modal urban air distribution described by CitationJaenicke (1993), and a mono-modal lognormal distribution of spheres with a mean diameter of 0.1 μ m, σ = 0.05 μ m and mr = 1.5. (c) Same as b, but for the GA retrieval of mr as a function of errors in the variance of the distributions.
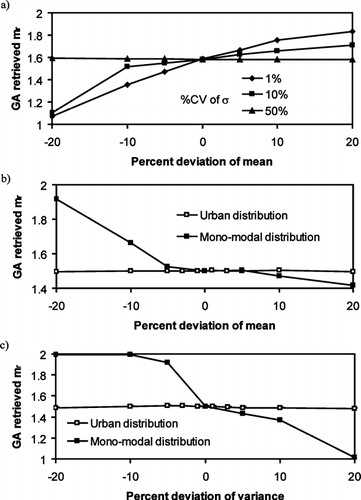
TABLE 1 GA retrieved real refractive indices from polar nephelometer measurements of PSL spheres
FIG. 6 (a) Nephelometer measured scattering properties for incident light polarized parallel (▴) and perpendicular (◊) to the scattering plane for (NH4)2SO4-H2O droplets when the relative humidity was about 60%. Error bars are based on the standard deviation of 10 separate measurements. The lines are the Mie-Lorenz scattering properties for spheres with incident light polarized parallel and perpendicular to the scattering plane and the GA determined real refractive index. (b) Same as b, but when the relative humidity was between 40 to 47%.
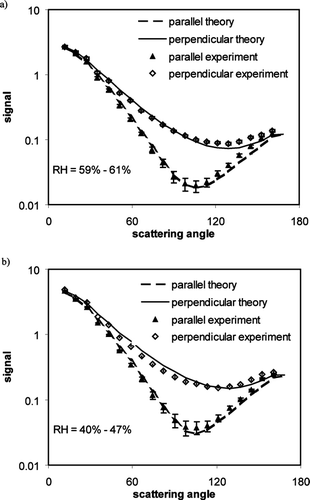
TABLE 2 GA retrieved real refractive index from the experimental scattering results measured from (NH4)2SO4-H2O droplets seen in
FIG. 7 (a) Nephelometer measured scattering properties for incident light polarized parallel (▴) and perpendicular (◊) to the scattering plane of SOA particles generated from the photochemical reaction of α -pinene. The lines are the GA determined scattering properties for homogenous spheres with mr = 1.43 fitted to the experimental results using the least squares method. (b) Representative TEM image of the SOA particles.
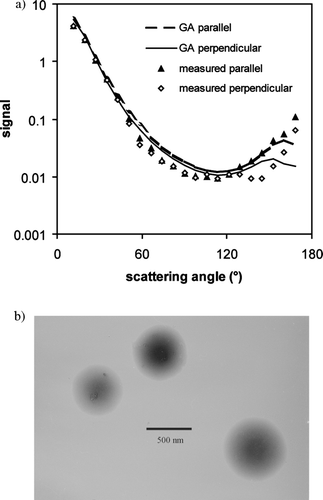