Figures & data
TABLE 1 Parameters for lognormal size distributionsFootnote a of (NH4)2SO4 and Ca(NO3)2 aerosols generated from solutions of varying concentration.
FIG. 1 TEM images of 535 nm PSL internally mixed with (NH4)2SO4 from 0.1 wt% solutions are shown in (a)–(c). Crystallites of (NH4)2SO4, designated by the arrows, are visible as islands on the surface of the PSLs. TEM images of 535 nm PSLs without (NH4)2SO4 coatings are shown in (d) and (e). The PSLs without (NH4)2SO4 are more spherical compared to the particles with (NH4)2SO4 although the uncoated particle surfaces do show some small “bumps” on the surface that may be due to impurities in the water.
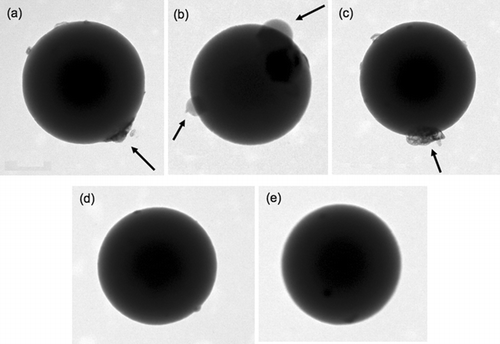
FIG. 2 Size distributions of 202, 356, and 535 nm PSLs shown mixed with various concentrations of aqueous (NH4)2SO4. Each size distribution displayed is a Gaussian fit to the measured SMPS distribution. The peak diameter of each distribution has been normalized to one. The distributions have been offset for clarity.
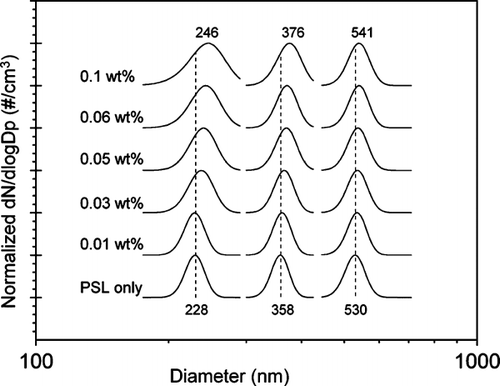
FIG. 3 A representative schematic of the particle shape and diameter used to determine the mass fraction of (NH4)2SO4 (fsolute) associated with each PSL sphere. A representation of the internally mixed particles based on the TEM images from is shown in (a), where solid crystallites of (NH4)2SO4 are formed on the PSL surface. When the particle is sized by the SMPS, the measured diameter is that of a sphere equivalent in volume to the internally mixed PSL particle. This diameter is represented by the dashed black line. In (b), the particle is assumed to have a uniform, crystalline layer of (NH4)2SO4 coating the PSL surface, where the thickness is the difference between the measured particle diameter, DP, and known PSL diameter, DPSL.
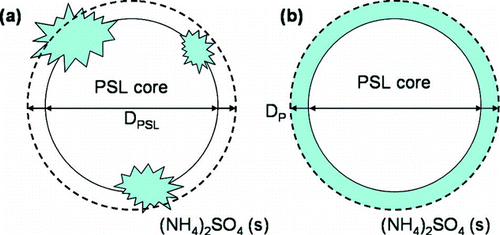
FIG. 4 (a) Calculated core CaCO3 diameter of 200 nm internally mixed CaCO3-Ca(NO3)2 particles generated from 0.01 (filled diamonds), 0.05 (open circles), and 0.1 (filled triangles) wt% Ca(NO3)2 solutions, shown as a function of atomizer droplet diameter (Dd). (b) Calculated mass fraction (fsolute) of Ca(NO3)2 in 200 nm internally mixed CaCO3-Ca(NO3)2 particles as a function of Dd. (c) Estimate of the frequency of occurrence of internally mixed 200 nm CaCO3-Ca(NO3)2 particles with a given fsolute size-selected from carbonate-nitrate mixtures of varying Ca(NO3)2 concentration.
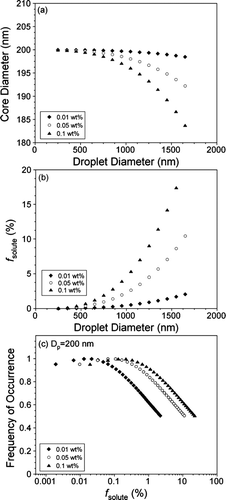
FIG. 5 CCN activity as a function of % supersaturation for 200 nm (a) pure CaCO3 (filled circles) and Ca(NO3)2 (open circles); (b) pure kaolinite (filled diamonds) and (NH4)2SO4 (open diamonds); (c) CaCO3 coated with 0.01 wt% (open triangles) and 0.05 wt% (filled triangles) Ca(NO3)2; and (d) kaolinite internally mixed with 0.01 wt% (open inverted triangles) and 0.02 wt% (filled inverted triangles) (NH4)2SO4. Each data point is the average of 2–10 measurements and the error bars represent the standard deviation of these multiple measurements. In (a) and (c), the solid black lines represent sigmoidal fits to the activity of pure Ca(NO3)2 and CaCO3, while the dashed black lines are sigmoidal fits to the internally mixed CaCO3 data. Similarly, the solid black lines in (b) and (d) represent sigmoidal fits to the activity of pure kaolinite and (NH4)2SO4 and the dashed black lines sigmoidal fits to the internally mixed kaolinite data. The Ca(NO3)2 and (NH4)2SO4 fits were calculated using a critical supersaturation value determined from Köhler theory. In each plot, the dashed gray line represents the 50% activation point. The data have all been normalized such that the maximum value of #CCN/#CN is one.
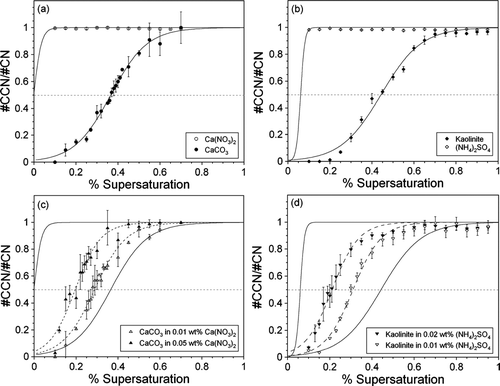
TABLE 2 Critical supersaturation values for 200 nm size-selected pure and internally mixed mineral dust components
FIG. 6 CCN activity at 0.3% SS for (a) Ca(NO3)2 (open circles) and CaCO3 (filled circles) and (b) CaCO3 internally mixed with 0.01 wt% (open triangles) and 0.05 wt% (filled triangles) Ca(NO3)2. The data are plotted as the fraction of particles that can nucleate a cloud (#CCN/#CN) as a function of mobility diameter. These particles represent partially processed mineral dust aerosol. Each data point represents the average of 2–14 measurements, and the error bars are equivalent to the standard deviation of these multiple measurements. The data have been normalized by multiplying each point by the reciprocal of the maximum activation. The dashed gray line indicates the critical diameter where 50% of the particles can activate a cloud. The solid black lines represent sigmoidal fits to the Ca(NO3)2 and CaCO3 data. For CaCO3, data points that are affected by multiple charging of the aerosol particles are not included in the sigmoidal fit. In (a), the points that have been excluded from the fit are shown in gray. The dashed black lines are sigmoidal fits to the internally mixed CaCO3-Ca(NO3)2 data.
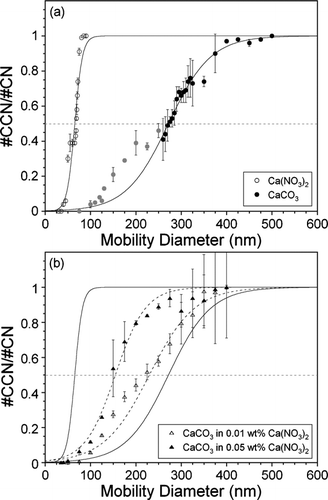
FIG. 7 Measured #CCN/#CN versus predicted #CCN/#CN for CaCO3 internally mixed with 0.01 wt% (open triangles) and 0.05 wt% (filled triangles) Ca(NO3)2 for diameters ranging from 150–400 nm at 0.3% SS. Moving across the x-axis the diameter of each data point increases by 25 nm. The predicted activities assume an external mixture and are based on the measured SMPS size distributions and CCN activity () of the individual components. The 200 nm point discussed in the text is indicated in the plot.
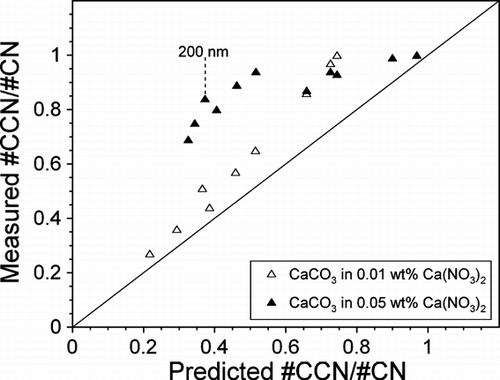
FIG. 8 Theoretical Sc, shown as a function of dry particle diameter, for Ca(NO3)2 (solid lines) and (NH4)2SO4 (dashed lines) droplets containing varying size insoluble cores. Sc has been calculated according to Köhler theory and assumes complete dissociation of Ca(NO3)2 and (NH4)2SO4. The series of lines shown are for a droplet containing no core and cores that represent 94.1, 97.0, and 99.7% of the total particle volume (corresponding to 98, 99, and 99.9% of the total particle diameter, respectively).
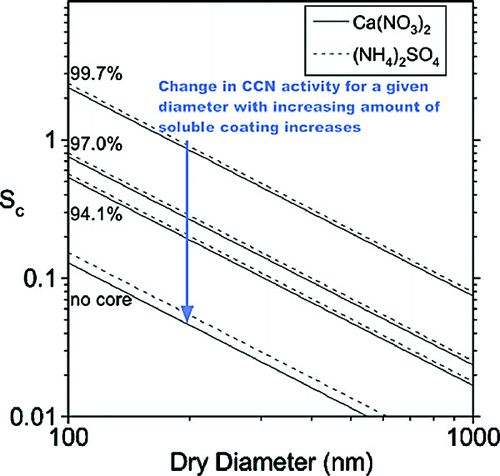