Figures & data
FIG. 2 An example of a measured pulse height spectrum, when PH-CPC is measuring with a high enough temperature difference to initiate homogeneous nucleation while detecting also large (>15 nm) particles (solid line). Homogeneously nucleated butanol droplets are seen in multichannel analyzer (MCA) channels 200–400 and aerosol particles in the MCA-channels 600–800. When calibration ions generated with a 60 MBq Am-241 source and sized with a Herrmann-DMA are directed to the PH-CPC, they show up at the MCA-channels 400–600 (circles).
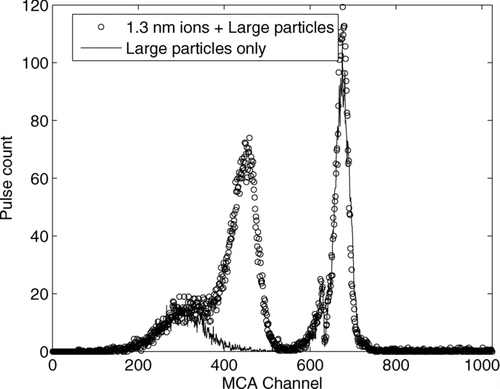
FIG. 3 The counting efficiency for positive charger generated ions and positive WOx –particles with a different saturator temperatures (Tsat) keeping the condenser temperature constant at 10°C. Capillary flow rate was 0.6 cm3s−1. At Tsat= 44°C and Tcond= 10°C even the smallest (∼1.2 nm) ions were detected with a counting efficiency of ∼5%. Transport efficiency was calculated according to Stolzenburg and McMurry (1992) and accounts for the losses in the aerosol capillary, CPC's inner inlet and 15 cm inlet tube.
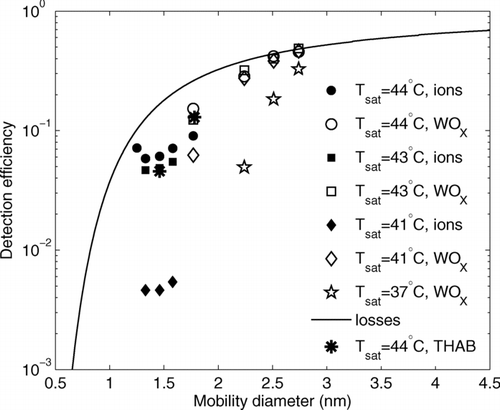
FIG. 4 Concentration of homogeneously nucleated droplets (a), and the counting efficiency for THAB dimer (1.78 nm, b) as a function of background concentration of 15 nm WOx -particles
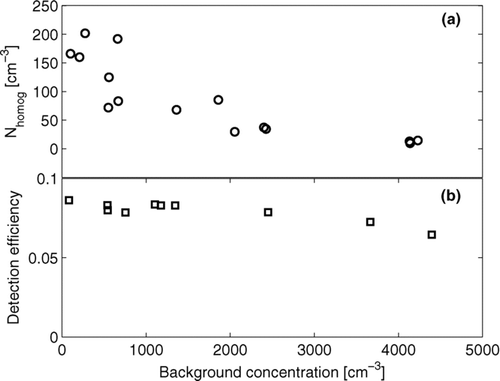
FIG. 5 Effect of charge polarity and particle composition on the counting efficiency. Negative WOx particles are counted more efficiently than positive ones. In the case of Am-241 charger generated ions, the opposite behavior is observed. The counting efficiency for the WOx particles is higher than for the NiCr particles and for the charger ions. Both the charge and the material dependency diminish towards larger particle sizes.
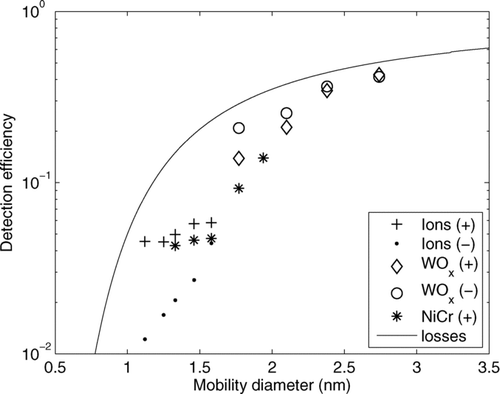
FIG. 6 Relation between the MCA peak channel and the particle diameter at different saturator temperatures (Tsat) for different positively charged calibration ions. Channel–diameter relation strongly depends on the supersaturation. Fitting to the data obtained with Tsat= 43°C is used in the data inversion.
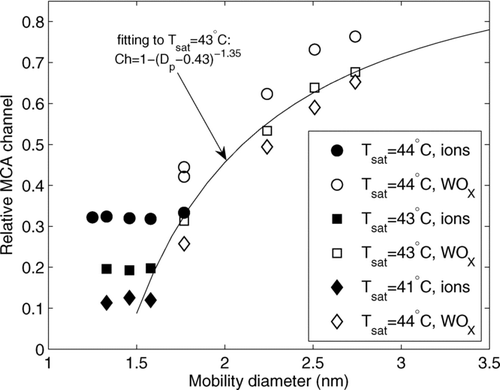
FIG. 7 Detection efficiency as a function of the MCA peak channel at different saturator temperatures for different positively charged calibration ions. The detection efficiency–channel relation is not strongly dependent on the supersaturation in this saturator temperature range.
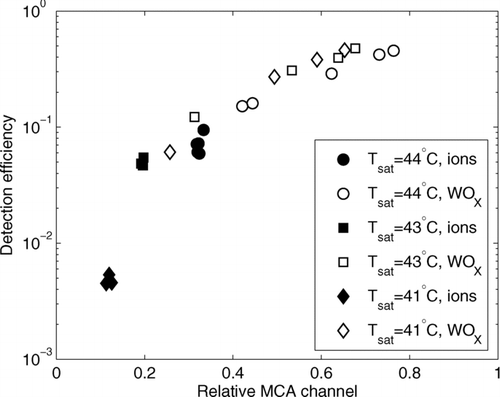
FIG. 8 Measured (solid lines) and modeled (dashed lines) kernel functions for monodisperse silver particles, and the modeled peak height (thick line). The concentrations are normalized by dividing with the particle concentration measured with the TSI electrometer. The measurements were done with the temperature settings Tsat= 43°C and Tcond= 10°C.
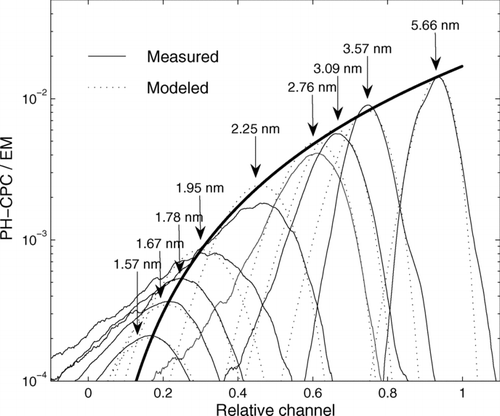
FIG. 9 Examples of the measured (solid lines) and filtered (squares) kernel functions for monodisperse ammonium sulfate particles.
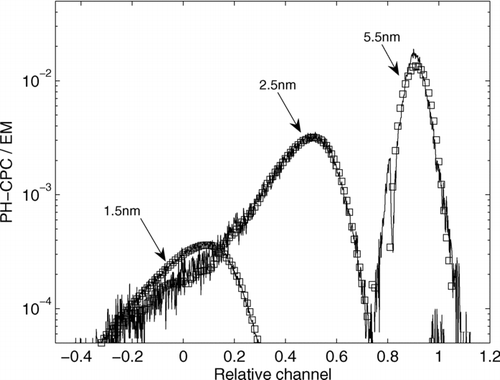
FIG. 10 The simulated lognormal size distributions (solid line) and the same distribution after converting it to MCA pulses, adding random noise and then inverting it back to real concentration using simulated kernel functions and pseudo inversion method (circles), or measured kernel functions and central solution from extreme value estimation (stars).
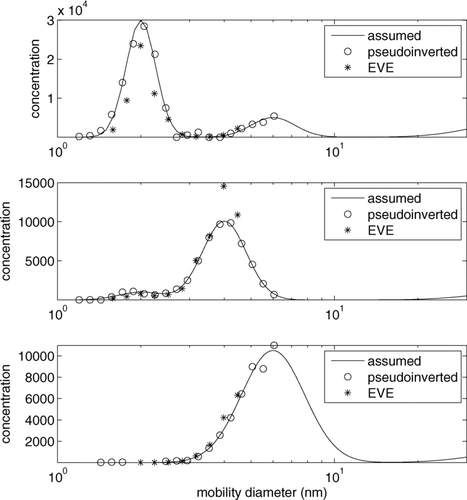