Figures & data
FIG. 1 Experimental setup of UDMA and APi-TOF in series. The radioactivity based 241Am aerosol charger is located directly at the ion-inlet of the UDMA. After the UDMA, the classified ions are detected by means of a custom built Faraday cup electrometer (FCE) and the APi-TOF. To study the dependency of the generated ions on the carrier gas, different experiments involving N2 (5.0), synthetic air (5.0) and filtered room air were performed.
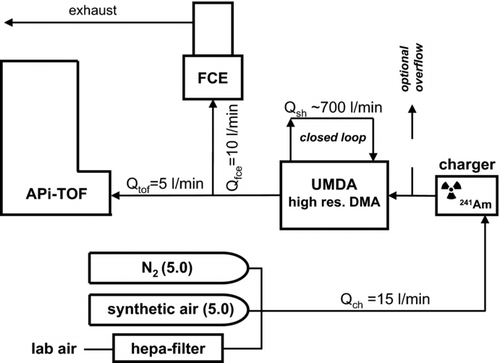
FIG. 2 (a) First number-size-distribution of ions generated in N2 with the dominant peak at 0.98 nm/2.09 cm2/Vs. (b) Corresponding mass spectrum of the classified ions with Z 1 = 2.09 cm2/Vs with dominating peaks: NO3 − (m/z integer 62 Th) and HNO3·NO3 − (m/z integer125 Th) peaks. Other strong signals originate from NO2 − (integer m/z 46) and H2O·NO3 − cluster (integer m/z 80).
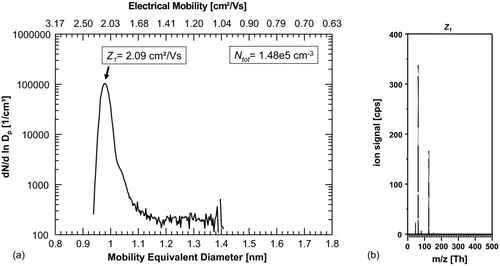
FIG. 3 (a) Number-size-distribution of ions generated in the charger after the baking-out. Three significant peaks can be found at 2.34, 1.42, and 1.08 cm2/Vs. The total number concentration of ions increases to 9.38 × 105 ions/cm3. (b) Corresponding mass spectra of the three significant size/mobility-peaks. Presently, we can only speculate about the actual cluster composition and give examples for possible configurations: the lowest selected mobility fraction (Z3 ) is dominated by integer m/z 213 (C9H10O2 .NO3 −), 425 (C9H10O2 .HNO3NO3 −), and 461 ((C9H10O2)2 .HCl.HNO3NO3 −). Z2 already shows a transition to smaller masses as I− (integer m/z 127) and NO3 − (integer m/z 62) arise Z1 is again dominated by HNO3·NO3 − (nitrate ion dimer, integer m/z 125) and NO3 − (integer m/z 62); other strong signals originate from lactic acid (m/z 89) and nitric acid trimer ((HNO3)2NO3 −, m/z 188).
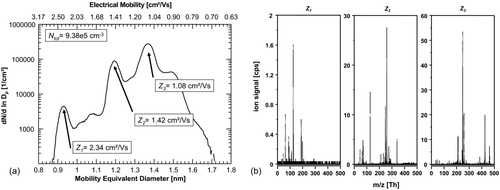
FIG. 4 (a) Number-size-distribution of negative ions produced in a 1:1-mixture of N2 (5.0) and synthetic air (5.0). The addition of synthetic air has only a minor effect on the overall pattern of the ion spectrum. Only the peak at 0.92 nm/2.34 cm2/Vs changed into a double-peak structure. (b) Corresponding mass spectra of the four significant size/mobility-peaks, showing a very similar pattern with the earlier N2 experiment.
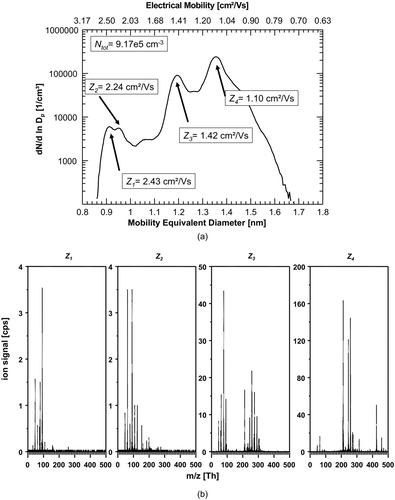
FIG. 5 (a) Number-size-distribution and of negative ions produced in synthetic air (5.0). A difference to the experiment using the 1:1-mixture of N2 (5.0) and synthetic air (5.0) can only be found in a slightly higher total ion number concentration of 1.08 × 106 ions/cm3. (b) The corresponding mass spectra of the four significant size/mobility-peaks using exclusively synthetic air also strongly resembles the situation of the 1:1-mixture of N2 (5.0) and synthetic air (5.0).
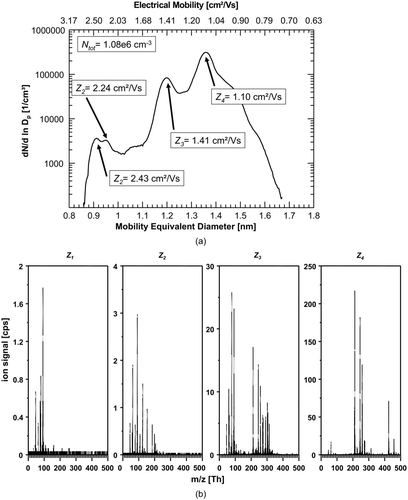
FIG. 6 (a) Number-size-distribution of negative ions produced in filtered (HEPA-filter), humid (rH∼30%) laboratory air. Again, a single mobility/size peak close to 1 nm with an electrical mobility of Z1 = 2.09 cm2/Vs is dominating the spectrum. (b) The corresponding mass spectra of the dominant size/mobility-peak again shows dominant signals of the nitrate ion (integer m/z 62) and its dimer (integer m/z 125). Apparently, by using a “contaminated” carrier gas, such as room air, the situation of the first experiments as presented in Figures 2a and b can be reproduced.
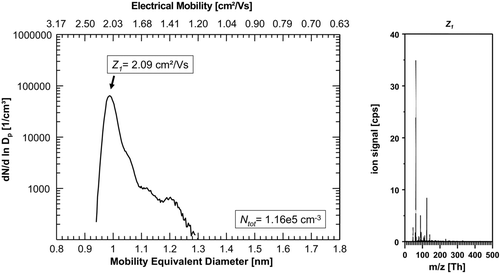
FIG. 7 Estimated size-dependent penetration efficiency of the high-resolution UDMA using a laminar diffusion deposition model with an effective length L eff of 22 m and a sample flow rate of 15 l/min. The shaded area corresponds to an assumed variation of 30% of the effective length L eff. In the typical size range of charger generated ions of about 1–1.5 nm, the penetration efficiency of the UDMA ranges from 0.5% for a 1 nm particle to 8.3% for a 1.5 nm particle; corresponding to a difference of a factor of 17.
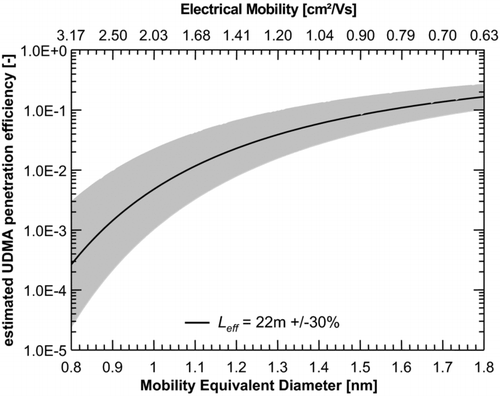
FIG. 8 Comparison of the total number concentration of ions during the course of the experiments. The black bars indicate the uncorrected data, as also presented in Figures 2–6. The grey bars account for diffusion losses, calculated by the size-dependent laminar diffusion deposition model. The error bars indicate the change in the corrected total number concentration by an assumed variation of the effective length L eff of ±30%. This way, the maximum difference of the concentrations reduces from a factor of 8 to a factor of 2.
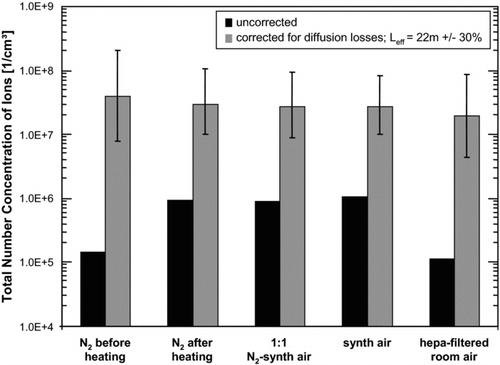
TABLE 1 Experimentally determined electrical mobility, reduced electrical mobility (Langevin rule), and mean m/z of selected mobility fractions of charger generated negative ions
FIG. 9 Reduced electrical mobility versus mass of ionic molecular clusters. Data derived during this study (black dots) is compared to data from Kilpatrick (Citation1971), Ku and Fernández de la Mora (Citation2009), Larriba et al. (Citation2011), a fit-function by Mäkelä et al. (Citation1996) to Kilpatrick's data and a power fit to the data by Kilpatrick (Citation1971), Ku and Fernández de la Mora (Citation2009), and Larriba et al. (Citation2011).
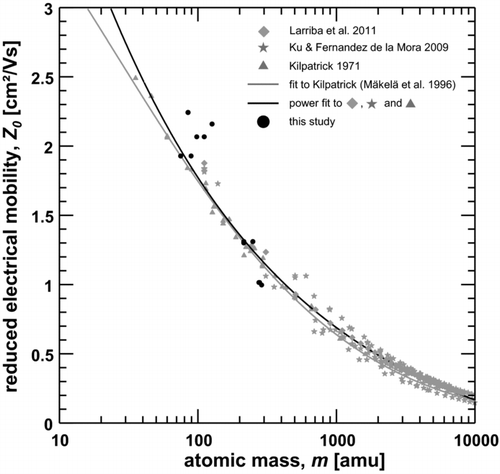