Figures & data
FIG. 1 Schematic of the combined thermal desorption aerosol gas chromatograph—aerosol mass spectrometer (TAG-AMS). TAG samples and AMS samples are alternately delivered to the high-resolution time-of-flight mass spectrometer. The TAG inlet delivers individual organic marker compounds and the AMS inlet delivers size-resolved fine aerosol that is separated into total organics, sulfate, nitrate, ammonium, and chloride fractions. Elemental ratios of O:C and H:C are attainable from both sample inlets using the high-resolution mass spectral capacity of the ToF-MS detector.
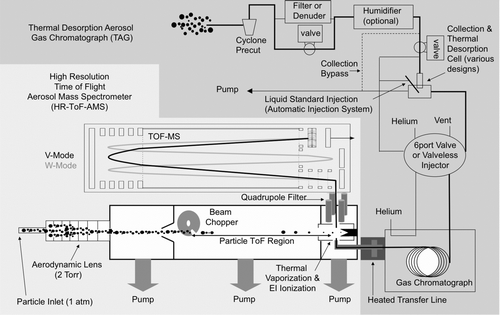
FIG. 2 Design and performance of a heated GC-MS interface. (a) The GC column is connected to a transfer line (coated stainless steel capillary) that travels through a heated copper block, delivering the sample to the ionization chamber prior to mass spectral detection. A quadrupole filter is used to deflect the large abundance of carrier helium gas prior to delivery to the ToF-MS. (b) Temperature profiles of heated transfer line system. For a 350°C set point temperature at the copper block, there is approximately a 40°C deviation as measured at the tip of the injector. Heat up time to reach stable operating temperature is about 20 min.
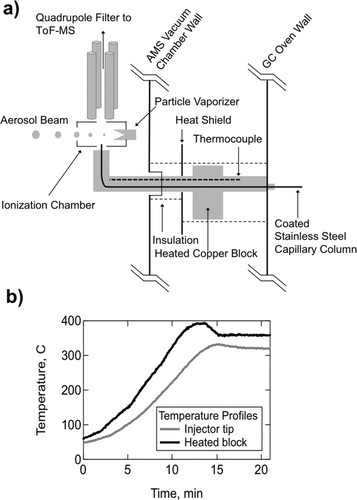
FIG. 3 Chromatograms of alkane/PAH standards. (a) 12.5 ng of each compound were first analyzed using a standalone TAG system (with quadrupole MS detection, Agilent 5973). (b) The same standard (12.5 ng of each species) was analyzed using the combined TAG-AMS system (with HR-ToF-MS, TofWerk). The same range of species are observed in both samples.
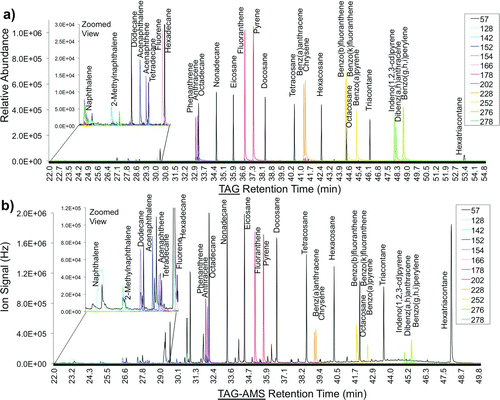
FIG. 4 TAG-AMS calibration curves for select chemical classes. TAG figures (a–e) display an integrated ion signal (counts) for each species (y-axis) per ng of species injected (x-axis): (a) alkanes, (b) PAHs, (c) carboxylic acids, (d) various acids, (e) various ketones, and AMS calibration using (f) ammonium nitrate (NH4NO3). Compounds were separated through TAG chromatography except for ammonium nitrate that was sampled through the AMS inlet.
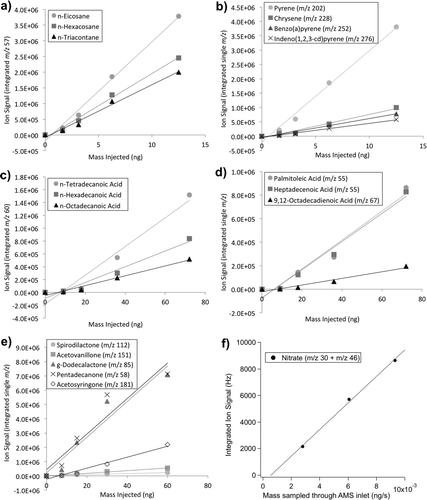
FIG. 5 Comparisons of oleic acid mass spectra. (a) Oleic acid mass spectra as obtained by the NIST reference library (black), by the TAG inlet on the TAG-AMS system (dark grey), and by the AMS inlet on the TAG-AMS system (light grey). Only select ions (largest contributors to total signal) are included. Individual ion responses have been normalized to the sum of all selected ions. (b) AMS and TAG mass spectra (MS) of oleic acid (y-axes) verses NIST MS reference (x-axis). Compared to TAG, here it is seen that AMS has a relatively higher response for smaller m/z's (41 and 55 labeled here), and a lower response larger m/z's (111, 123, and 264 shown here).
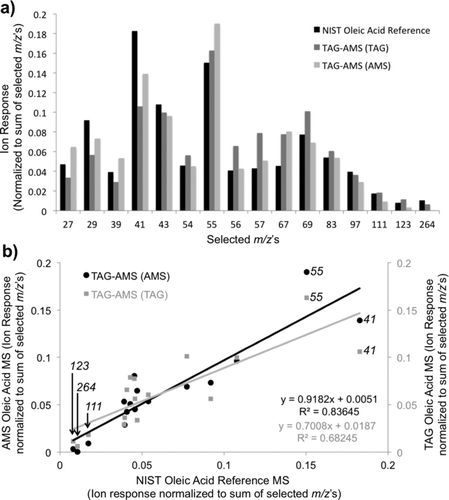
FIG. 6 TAG chromatogram from Aerodyne Research Inc. laboratory air (collected overnight on 15 January 2009). (a) Single ion chromatograms for unit mass m/z 43 and m/z 57, (b) single high-resolution ion chromatograms for C2H3O, C3H7, C3H5O, and C4H9 shown to separate the hydrocarbon peaks from oxygenated peaks, (c) example high-resolution mass spectra focused around the m/z 43 and m/z 57 range for an early retention period in the chromatogram, showing both oxidized and hydrocarbon signals, and (d) example high-resolution mass spectra focused around the m/z 43 and m/z 57 range for a late retention period in the chromatogram, showing the dominance of the hydrocarbon signal. (e) A zoomed image of the early retention time section of the high-resolution ion chromatograms shown in (b). Here, it can be seen that both oxygenated ions and hydrocarbon ions contribute to species observed in this section of the sample.
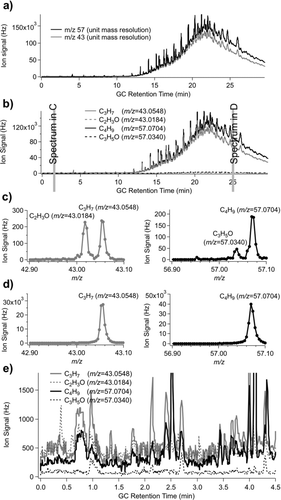
FIG. 7 TAG chromatogram from Aerodyne Research Inc. laboratory air (collected overnight on 15 January 2009). (a) Elemental analysis across chromatogram to show O:C ratios and H:C ratios as a function of chromatography volatility. Low-volatility oxygenated OA does not transfer efficiently through a 30 m GC column, partially explaining the decreased O:C ratio at higher column temperatures. (b) van Krevelen diagram outlining the H:C ratio vs. O:C ratio color coded by GC retention time. Maximum O:C ratios are reached around retention times = 8 min, and minimum O:C ratios are reached for retention times >16 min. (c) TAG total ion signal displayed with the same retention time color code as (b).
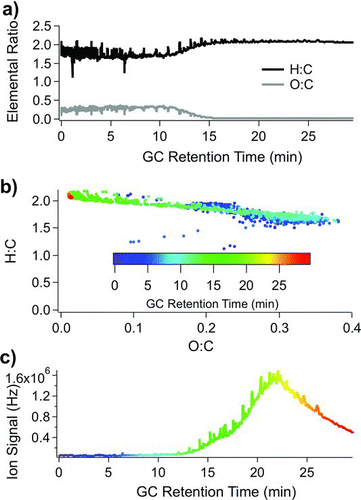