Figures & data
![FIG. 1. Illustration of DMA sizing error. When a voltage V1 is applied to the DMA, particles from the tail of the aerosol population with mobilities in the common region under the original distribution f0(Z) and the transfer function Ω(V1, Z) are classified/detected. A mobility Z1(V1), given by EquationEquation (1)[1] , is assigned to these particles in spite that there are no particles with such a high mobility in the aerosol population.](/cms/asset/7661f400-0970-4b0d-93b5-dc7cafc2075e/uast_a_973931_f0001_oc.jpg)
![FIG. 2. Example of particle classification with a single DMA. f0 is the mobility distribution of the aerosol. Ωnd is the ideal transfer function for non-diffusive particles; Ω is the transfer function calculated by: Stolzenburg's model (solid line), MonteCarlo simulation (dots), and lognormal approximation (8) (dashed line); f1 is the fraction of particles of mobility Z classified at voltage V1 divided by dlnZ, calculated from EquationEquation (4)[4] using the transfer function calculated by: Stolzenburg's model (solid line), MonteCarlo simulation (dots), and lognormal approximation (8) (dashed line). The theoretical mobility directly inferred from the applied voltage and EquationEquation (1)[1] is denoted as Z1; the true mobility of the classified particles is shown as Z1, true. Data obtained for the Nano-DMA of Chen et al. (Citation1998) operated at Qa/Qsh = 2/20 (in lpm) and V1 = 12.6 V; aerosol mobility distribution: Zg0 = 0.25cm2/Vs (Dpg = 2.9 nm); σg0 = 1.2.](/cms/asset/1d661fc5-9cae-49fd-a7ec-4ba9e13a9466/uast_a_973931_f0002_oc.jpg)
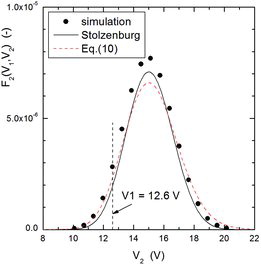
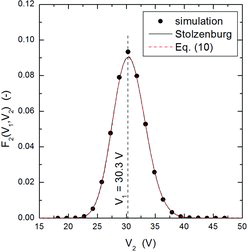
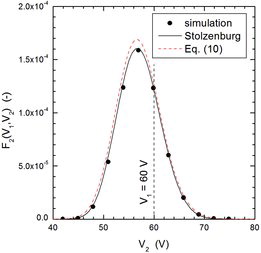
![FIG. 6. Comparison between the true mobility of particles classified by DMA-1 calculated from MonteCarlo simulations by two approaches, using (i) EquationEquation (5)[5] (y-axis), and (ii) EquationEquations (2)[2] and Equation(7)[7] (x-axis). Data obtained for the Nano-DMA (triangles: Qa/Qsh = 1/10; squares: 2/20) with Zg0 = 0.25 cm2/Vs and σg0 between 1.1 and 1.8; and the Vienna type DMA (circles) operated at Qa/Qsh = 2/20, with Zg0 = 0.20 cm2/Vs and σg0 between 1.2 and 2.0.](/cms/asset/fcd849bc-00d8-4cb1-b2db-ca0959475f27/uast_a_973931_f0006_oc.jpg)
![FIG. 7. Comparison between the mobility calculated with EquationEquation (1)[1] and the true mobility of particles classified with a DMA. Nano-DMA operated at a flow rate ratio of 1/10. The MonteCarlo simulation results are represented by open symbols; those obtained using the rigorous (i.e., not the lognormal approximation) transfer function of Stolzenburg are plotted with solid symbols. The solid curves were calculated using EquationEquation (9)[9] . The aerosol had a mean geometric mobility of 0.25 cm2/Vs (mobility-equivalent particle diameter of 2.9 nm) and the geometric standard deviations shown in the plot.](/cms/asset/0fcc0cd2-0d8a-4911-bef9-fb151bab1e2e/uast_a_973931_f0007_oc.jpg)
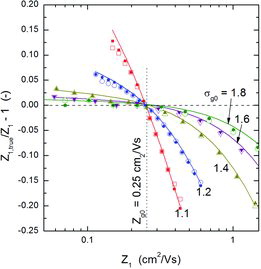
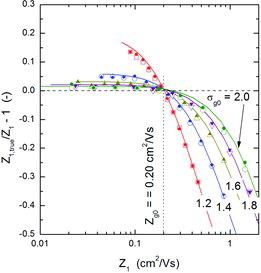
Register now or learn more
Free access
Please note: Selecting permissions does not provide access to the full text of the article, please see our help page How do I view content?
To request a reprint or corporate permissions for this article, please click on the relevant link below:
Please note: Selecting permissions does not provide access to the full text of the article, please see our help page How do I view content?
Obtain permissions instantly via Rightslink by clicking on the button below:
If you are unable to obtain permissions via Rightslink, please complete and submit this Permissions form. For more information, please visit our Permissions help page.
People also read lists articles that other readers of this article have read.
Recommended articles lists articles that we recommend and is powered by our AI driven recommendation engine.
Cited by lists all citing articles based on Crossref citations.
Articles with the Crossref icon will open in a new tab.