Figures & data
Figure 1. (a) Example data for PAS microphone response, using the PA cell design of Lack et al. (Citation2006), over time during airborne measurements aboard the UK research aircraft (FAAM BAe-146), including measurements for sampling ambient aerosol particles and for where the sample was passed through a HEPA filter (background). Measurements were made above the South East Atlantic Ocean during flight C043 of the CLARIFY-2017 field campaign based at Ascension Island (Zuidema et al. Citation2016). For comparison, we show the variation in pressure associated with changes in altitude over the range 0–7.5 km. The plot also shows the interpolated pressure-dependent background, using a quadratic polynomial to relate the background response to pressure. (b) The standard error in PAS-measured αabs associated with uncertainty in the interpolated background microphone response.
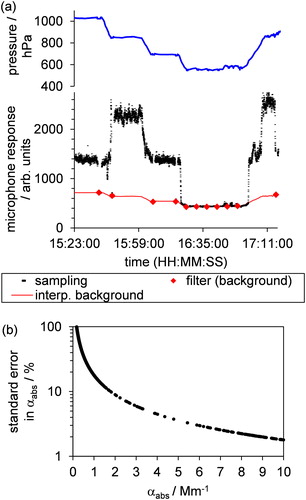
Figure 2. (a) The meshed geometry for the two-resonator cell with flat windows including two reflection symmetry planes; one parallel to the z-x plane through the center of the cell (longitudinal mirror plane) and one parallel to the z-y plane (transverse mirror plane). (b) The meshed geometry for the Brewster window cell. (c) Labeled dimensions for the Brewster window cell, with perspectives along the longitudinal and transverse mirror planes. provides values for these dimensions. (d) For the Brewster window cell, the highlighted volumes indicate regions where periodic sample or window heating was applied.
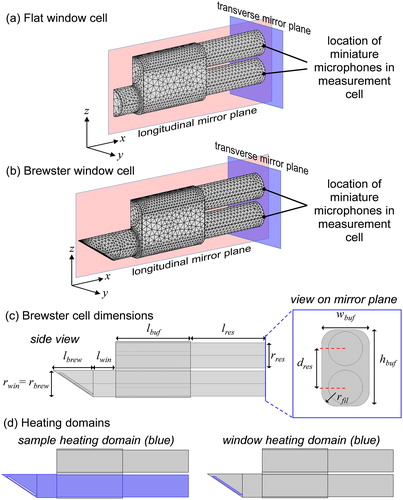
Table 1. Geometric parameters for the two-resonator PA cell.
Figure 3. Comparison of the eigenmode pressure distributions for the two-resonator cell with either (a) flat windows or (b) Brewster-angled windows.
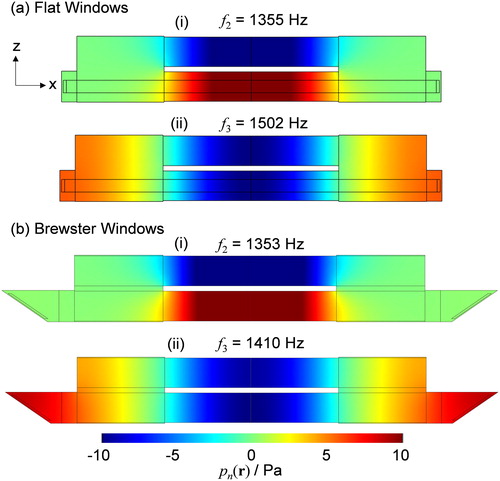
Figure 4. FEM predictions for the flat window cell of the frequency-dependent pressure responses ,
and
. Simulations are shown for the cases of (a) sample heating (αsample = 5 Mm−1) and (b) window heating (αwin = 0.1653 m−1). We compare the sample heating simulations to measured data using speaker excitation, with measurements representing the mean response over five PA spectrometers.
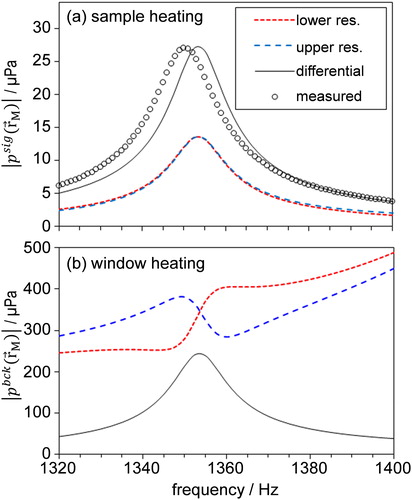
Figure 5. FEM predictions of microphone response for the Brewster window cell compared with measured data. (a) Simulations of for the individual microphone and differential responses compared to measured differential operation data using speaker excitation. (b),(c),(d) Predictions of
(window heating) with the measured IAbck for detection by the lower microphone, upper microphone, and differential response, respectively. Measured data are shown for four separate PA spectrometers using 405 nm (two spectrometers), 514 nm, or 658 nm laser excitation. The measured IAbck has been normalized to scale from 0 to 1. The lines for the measured IA series are to guide the eye only.
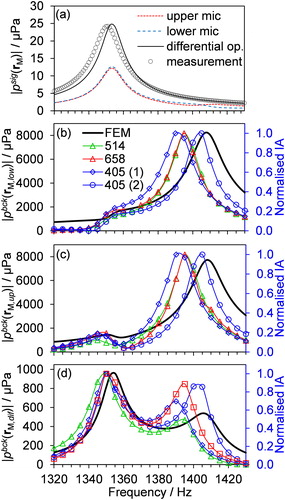
Figure 6. The eigenmode pressure distributions pn() for (a) the ring mode, and (b) the longitudinal mode with varying lbuf. The color scale range has been expanded to emphasize important variations in pn(
) with changing lbuf. In particular, the variation in pn(
) within the window volumes for the ring mode and the eigenmode pressure in the two resonator pipes for the longitudinal mode is emphasized.
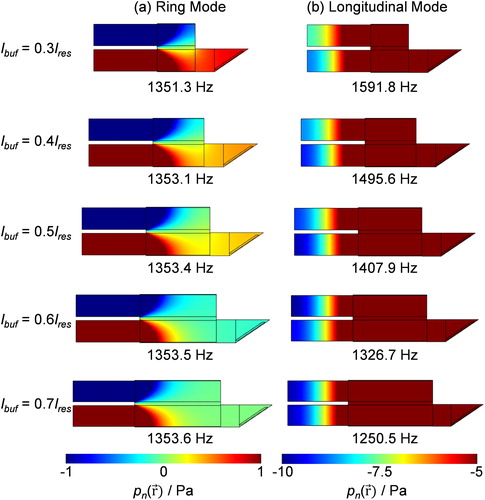
Figure 7. The FEM-predicted frequency dependence of (a) |psig(| and (b) |pbck(
| for values of lbuf in the range 0.1 lres – 0.7 lres in 0.1 lres intervals. The legend indicates the
ratio for different series. (c) The variation in SBR with lbuf.
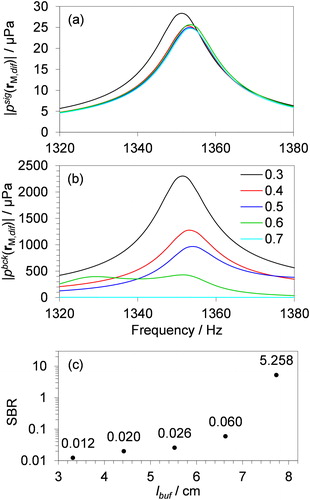
Figure 8. The variation in (a) |psig( M,dif, ωn)|, (b) |pbck(
M,dif, ωn)|, and (c) SBR with wbuf over the range 2.5–4.5 cm. Different data series are shown for the range of hbuf input to FEM simulations, from 4.5 cm to 6.0 cm in 0.5 cm steps. Lines are to guide the eye only.
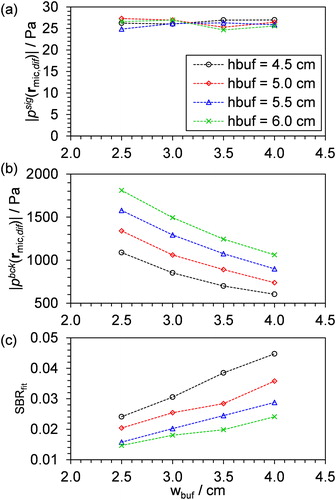
Figure 9. (a) The predicted SBR with variation in lbuf/lres for the candidate two-resonator cell for which the total cell length is constrained to 22 cm, compared with the SBR variations presented in . (b) Comparison of the predicted frequency-dependent differential amplified microphone responses for sample heating for the original (blue curve) and optimized (red curve) PA cells. (c) Same as (b) but for window heating excitation. The ring mode eigenfrequencies for the current (dashed blue line) and optimized (dashed red line) cells are indicated.
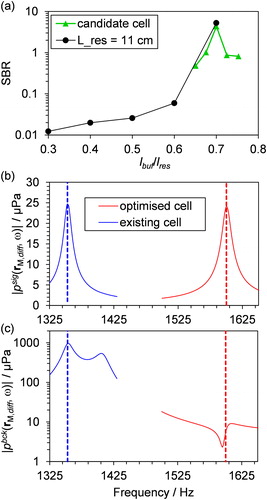
Table 2. Geometric parameters describing the optimized two-resonator PA cell.
Figure 10. (a) For laser heating of the Brewster windows, the measured IA at the ring mode eigenfrequency for the original (old) and optimized (new) cells for laser wavelengths of 405 and 658 nm. The mean IA for ambient noise when the laser is off is also shown. (b) A time series in the measured IA for the optimized cell when the 658-nm laser is on or off. An indicative scale is shown on the right axis for absorption coefficient based on calibration of the cell using O3.
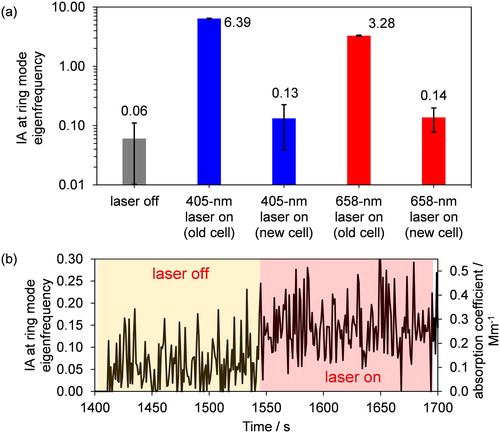
Figure 11. Comparison of FEM predictions of |pbck( M, ω)| with measurements of microphone response (IAbck) for the optimized cell for (a) lower and upper microphone responses, and (b) differential amplifier response. Error bars in (a) and (b) indicate one standard deviation in the measured IAbck for 30 s of measurements.
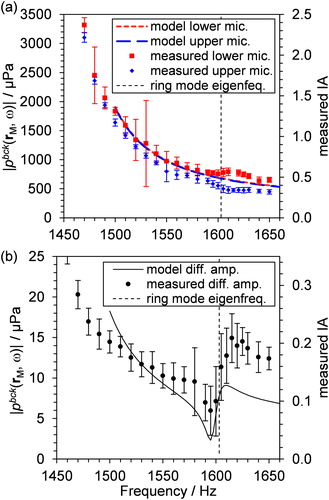
Supplemental Material
Download PDF (1.1 MB)Data availability
For data related to this paper, please contact Michael I. Cotterell ([email protected]).