Figures & data
Figure 1. (a) Schematic illustration of the RF-powered, flow-through, atmospheric-pressure plasma reactor with downstream DC bias used to study aerosol nanoparticle charging. (b) Charge fraction of aerosolized MgSO4 nanoparticles measured after plasma reactor as a function of particle diameter and different DC biases. The collection efficiency of aerosolized NaCl particles measured after an ESP reported by Zhuang et al. (Citation2000) is shown for comparison. (c) Charge fraction of aerosolized MgSO4 nanoparticles with 25 and 45 nm diameter after plasma reactor as a function of DC bias polarity.
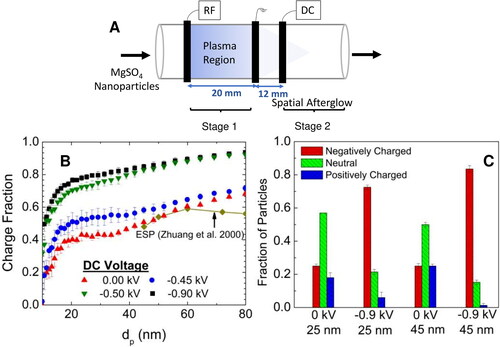
Figure 2. Calculations of characteristic times for positive ions colliding with and being lost to the reactor walls and colliding with and neutralizing negatively charged nanoparticles in the spatial afterglow as a function of the DC bias voltage.
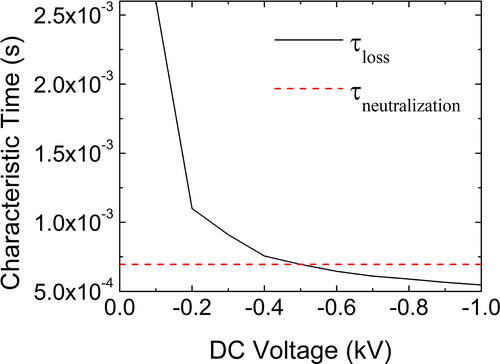
Figure 3. Schematic illustration of the two key particle charging regimes that exist in a RF, flow-through, atmospheric-pressure plasma reactor with downstream DC bias: one, the main plasma volume where nanoparticles are predominantly charged negatively by the higher mobility electrons, and two, the spatial afterglow where positive ions neutralize and/or charge positively the negatively charged particles when the DC bias voltage is less than a critical voltage, or where positive ions are removed and the negatively charged particles are preserved above a critical voltage.
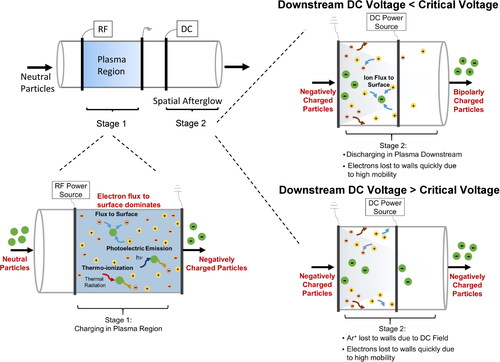