Figures & data
Figure 2. Aerosol chamber schematic. The test system consisted of a cylindrical 67.5-L polycarbonate chamber supplied with room temperature humidity controlled air. Aerosol generation used an air assist nozzle attached to the top of the chamber that produced aerosol with an initial VMD of 6.3 µm and a GSD of 1.5. The generated aerosols were sampled from ports located at the distal end of the chamber using two 25-mm PTFE filters and an aerodynamic particle sizer (APS), all flowing at 5 L/min. The final equilibrated particle size distribution measured by the APS had an MMAD of 1.3–1.5 µm and a GSD of 1.5–1.6. An additional 40 L/min of exhaust flow was pulled from a separate HEPA-filtered port, resulting in a total exhaust flow of 55 L/min.
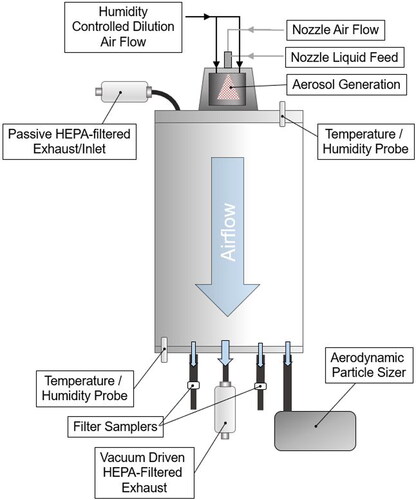
Figure 3. Schematic of test system to evaluate losses of viral infectivity associated with the aerosol nozzle. To isolate losses during aerosol generation, the output of the nozzle used for aerosol generation was collected directly into an impinger containing ten mL of collection medium. For testing with virus, the collection medium was gMEM without FBS. Aerosol was generated for 45 s for each test. Airflow through the nozzle during the test resulted in evaporation of <10% of the collection medium in the impinger. Initial testing using a fluorescent tracer demonstrated complete recovery of material sprayed into the collection medium, with an average recovery of 101.0 ± 2.5% using PBS as a collection medium.
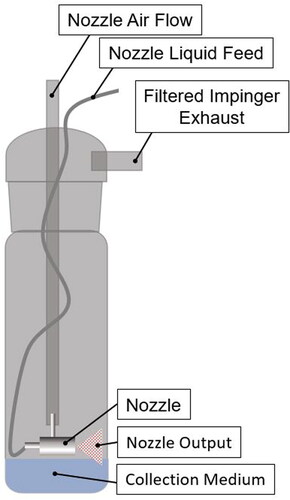
Figure 4. Losses of infectivity of different isolates of SARS-CoV-2 in aerosol particles post-evaporation. Losses of viral infectivity for different variants of SARS-CoV-2, normalized for physical losses in the test system, are shown. No significant differences were observed between any of the isolates or suspension media evaluated (P > 0.35 for all comparisons). Squares represent the losses for each individual test, estimated as the average of the two PTFE filters sampling at 5 L/min. Lines represent the mean ± one standard deviation.
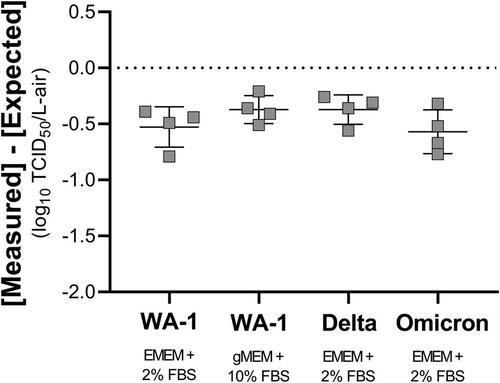
Table 1. Summary of infectivity losses and particle size statistics for different isolates of SARS-CoV-2 in aerosol particles post-evaporation.
Figure 5. Comparison of losses of SARS-CoV-2 infectivity in aerosol particle pre- and post-evaporation. Average losses of viral infectivity for all isolates of aerosolized SARS-CoV-2 measured pre-evaporation, following collection in a liquid impinger, and post-evaporation, following collection using PTFE filters, are shown. The average loss of viral infectivity measured pre-evaporation was −0.04 ± 0.25 log10 TCID50, which was not significantly different than zero (P = 0.41). The average loss of viral infectivity measured post-evaporation, after normalizing for physical losses, was −0.46 ± 0.17 log10 TCID50/L-air, significantly greater than the pooled value measured for the aerosol pre-evaporation (P < 0.0001).
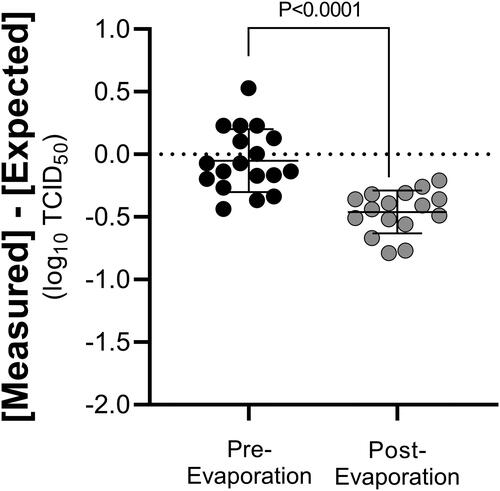