Figures & data
Table 1. List of the publications using the BB06-h, BB06-l, and CC90 for BC refractive index.
Figure 1. Schematic diagram of the complex amplitude sensor for waterborne particles. A linearly polarized 2 mW He-Ne laser with λ = 0.633 μm was used for generating high-wavefront quality Gaussian laser beam. An optical isolator was used to prevent laser instability due to back reflections. Each pair of rotatable half-wave plates (HWPs) with polarization beam splitters (PBSs) was used to split the beam with a controlled power ratio. The beam optics in the s- and l-channels are configured to quantify the complex forward-scattering amplitude of the sub- and super-micron particle size range, respectively. Table S1 lists the models and manufacturers of all the optical components in this schematic.
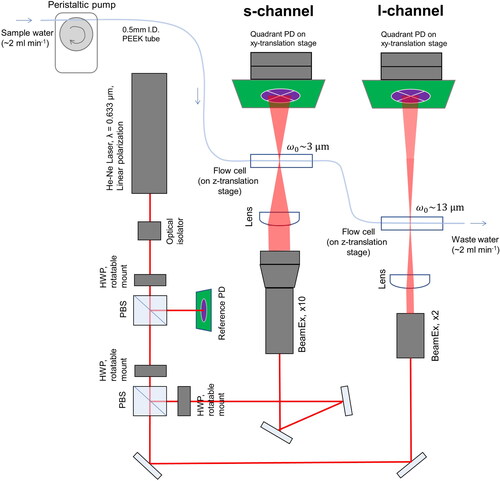
Figure 2. Examples of the geometric arrangement of spherical monomers in the AGGREGATE and SPHPACK models. (a) AGGREGATE with fractal dimension 2.0, (b) AGGREGATE with fractal dimension 2.5, (c) SPHPACK with packing density 0.05, (d) SPHPACK with packing density 0.30. The number of monomers Npp is 1024 in each example. The length unit of each Cartesian coordinate is the monomer radius.
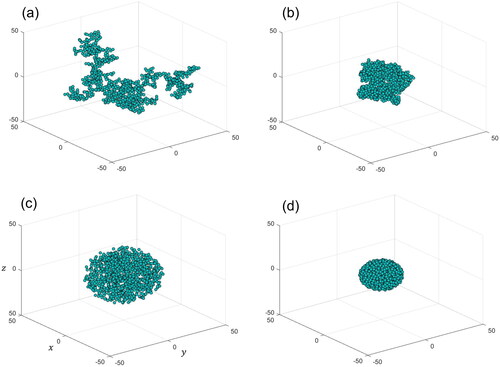
Table 2. List of the particle shape models and their parameters (rv, mr, mi, θs).
Figure 3. Flow chart of the computational and data processing procedure in our method for constraining the refractive index of ambient BC from the complex forward-scattering amplitude measurements. The frame color shows either of data, forward model, or Bayesian probability as illustrated in the lower-left part of the Figure.
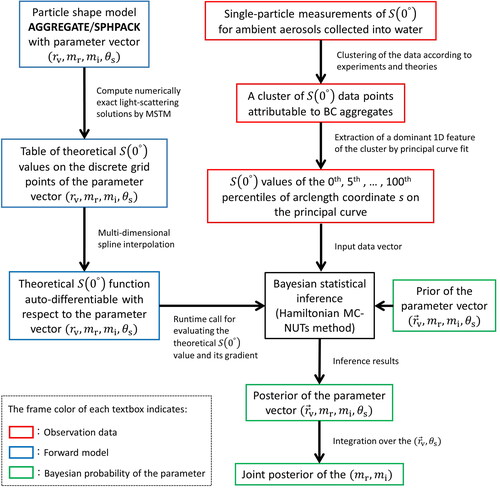
Figure 4. Typical examples of transmission electron microscope (TEM) images of laboratory powder samples. (a) Fullerene soot, (b) BC aggregate in the Vehicle exhaust particulates, (c) Hematite-KJ, and (d) Hematite-TD. The TEM images were obtained using a 120-kV TEM (JEM-1400, JEOL).
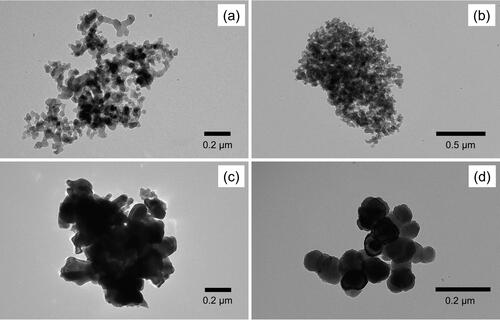
Table 3. List of the laboratory test samples.
Figure 5. Typical examples of transmission electron microscope (TEM) images of ambient aerosols collected by an aerosol-impactor sampler installed on the research vessel SHINSEI MARU. Each panel shows samples collected around 12:00 local time on (a) July 27th, (b) July 28th, (c) July 30th, and (d) August 1st. Red arrows indicate individual BC aggregates, most of which were mixed with sulfate (green arrows) and/or organics (light blue arrows). The TEM images were obtained using a 120-kV TEM (JEM-1400, JEOL).
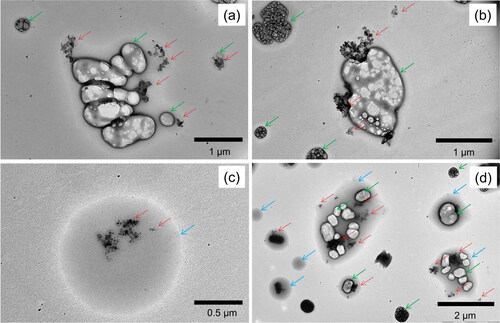
Figure 6. Scatterplot of the complex forward-scattering amplitude obtained for each of the laboratory powder samples suspended in water. Black dots show raw single particle S(0°) data. The red-filled circles show the 0th, 5th, …, and 100th percentiles of the arclength coordinate of the principal curve. These 21 data points were used as the observation data vector for Bayesian inference. The red circles are concentrated in proportion to the local density of raw data points.
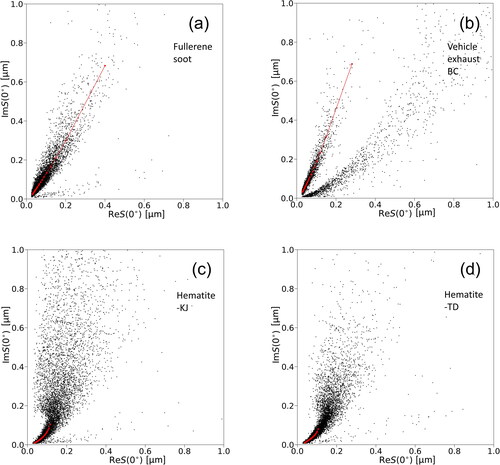
Figure 7. Same plots as but for atmospheric water-insoluble aerosols collected into water on each of the 6 observation days. Principal curve fit was applied to the linear-shape cluster of data points with ImS(0°)/ReS(0°) > ∼1, which is attributable to BC aggregates.
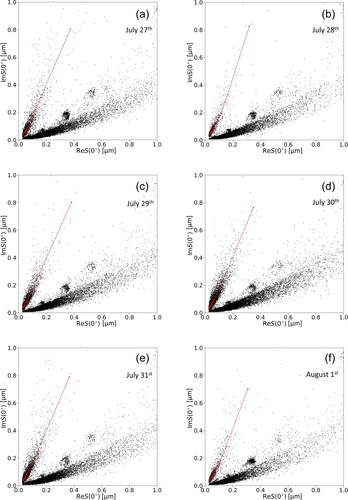
Figure 8. Posterior of real and imaginary parts of complex refractive index (mr, mi) for each laboratory sample derived from the data shown in . (a) Fullerene soot, (b) Vehicle exhaust BC, (c) Hematite-KJ, (d) Hematite-TD. The three-level contour shows the 90%, 50%, and 10% highest density credibility regions for each of the AGGREGATE (green) and SPHPACK (blue) models. Marginalized density distributions of mr and mi are also shown along horizontal and vertical axes, respectively. In each panel, the BB06-l and -h values were also shown by gray-filled circles for comparison. In panels (a) and (b), the SPHPACK is more realistic shape model than the AGGREGATE for the reasons explained in the main text.
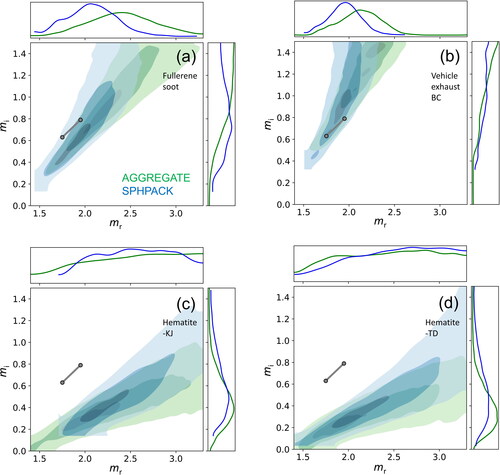
Figure 9. Same plot as but for atmospheric BC results derived from the data shown in . In each panel, the BB06-l and -h values were also shown by gray-filled circles for comparison. The dashed lines indicate the boundary of our suggested plausible (mr, mi) domain for atmospheric BC, which approximate the 90% highest density credibility regions. The SPHPACK is more realistic shape model than the AGGREGATE for the reasons explained in the main text.
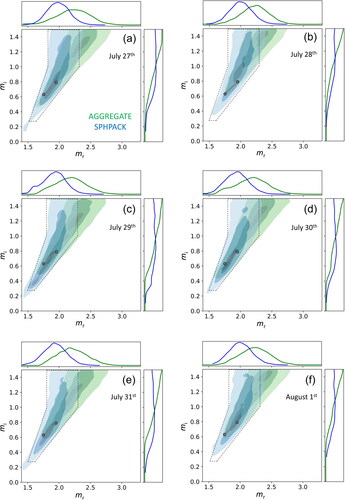
Figure 10. Joint density plots of the posteriors of real and imaginary parts of refractive index, mr, mi, and the two volume-equivalent radii corresponding to the 95th and 100th percentiles of arclength coordinate of the principal curve, rv95, rv100 for atmospheric BC sampled on August 1st. The three-level contour shows the 90%, 50%, and 10% highest density credibility regions in each of the AGGREGATE (green) and SPHPACK (blue) models. The SPHPACK is more realistic shape model than the AGGREGATE for the reasons explained in the main text.
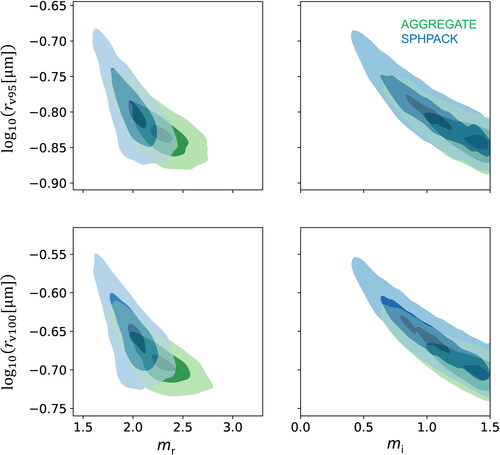
Figure 11. Illustrating the three-different (mr, mi) domains for complex refractive index of BC: 1) a domain consistent with measured S(0°) for atmospheric BC (enclosed by black-dashed lines), 2) a domain consistent with measured MAC for various flame-generated BCs (red-filled area), and 3) a domain supported by both MAC- and S(0°)-measurements. The conventional assumptions BB06-l (1.75 + 0.63i) and -h (1.95 + 0.79i) (gray filled circles), and our recommendable value for practical uses 1.95 + 0.96i (blue-filled circle) are also plotted.
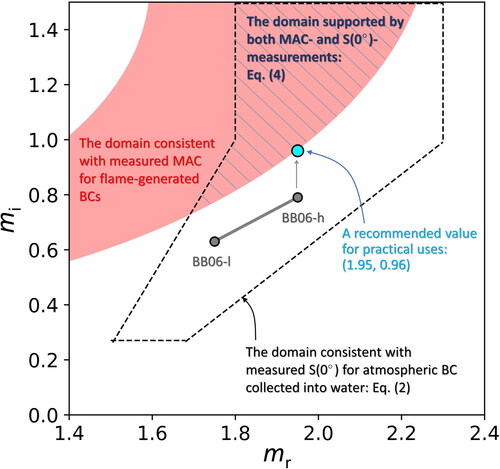
Supplemental Material
Download PDF (4.6 MB)Code availability
The originally developed Python module used for auto-differentiable multidimensional spline interpolation of gridded data is available from: https://github.com/nmoteki/ndimsplinejax. The code used for generating fractal-like aggregates of spheres is available from https://github.com/nmoteki/aggregate_generator. Other codes used for this study are available from the corresponding author upon reasonable request.
Data availability statement
Data generated for and are available from: https://doi.org/10.5281/zenodo.7478889.