Figures & data
Figure 1. The rotating frame of reference, with the -axis chosen horizontally due East, the
-axis horizontally due North and the
-axis upward.
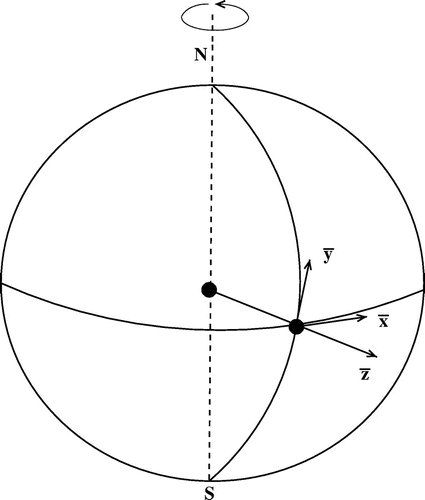
Figure 2. Sketch of the cross-section of the fluid domain at a fixed latitude: the thermocline separates the two layers of different densities, the lower boundary is a flat rigid bed, while the upper boundary is a free surface of elevation
. The coupled surface and internal waves propagate at the same speed, with the amplitude of the oscillations of the thermocline typically considerably larger.
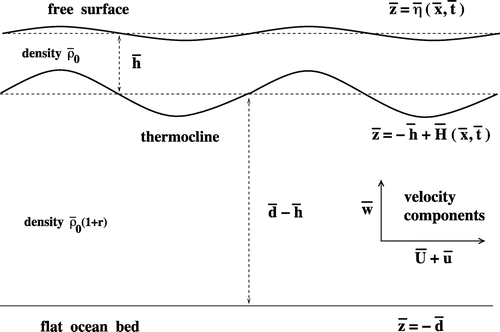
Figure 3. An example of the underlying current profile in the absence of waves, using the mean depth of the thermocline as the length scale. We distinguish four regions: (i) a subsurface layer in which a current of constant negative vorticity captures the salient features represented by a westward surface wind-induced drift, below which resides the EUC in the form of an eastward jet; (ii) the layer
in which the core of the EUC is represented by a uniform eastward current; (iii) the layer
in which a current of positive vorticity enables the transition to the practically motionless layer beneath it; (iv) the abyssal layer
, not depicted in full, where we take the water to be stationary. The thermocline
is located in the second layer (the core of the EUC). Typical values are
,
and
, corresponding to a thermocline depth of
m, a subsurface layer of depth
m where the effects of wind are noticeable, and an abyssal layer of practically motionless water beneath
m, extending down to the ocean bed, located at a depth of
km.
![Figure 3. An example of the underlying current profile in the absence of waves, using the mean depth of the thermocline as the length scale. We distinguish four regions: (i) a subsurface layer z∈[-1+l,0] in which a current of constant negative vorticity captures the salient features represented by a westward surface wind-induced drift, below which resides the EUC in the form of an eastward jet; (ii) the layer z∈[-1-m,-1+l] in which the core of the EUC is represented by a uniform eastward current; (iii) the layer z∈[-n,-1-m] in which a current of positive vorticity enables the transition to the practically motionless layer beneath it; (iv) the abyssal layer z∈[-d,-n], not depicted in full, where we take the water to be stationary. The thermocline z=-1 is located in the second layer (the core of the EUC). Typical values are l=m=1/3, n=2 and d=33, corresponding to a thermocline depth of 120 m, a subsurface layer of depth 80 m where the effects of wind are noticeable, and an abyssal layer of practically motionless water beneath 240 m, extending down to the ocean bed, located at a depth of 4 km.](/cms/asset/471ad3bd-d7c8-4129-bd92-9c6a0d5e56ff/ggaf_a_1066785_f0003_b.gif)
Figure 5. The direction of propagation has an effect on the coupling of the surface and internal waves: (a) The eastward propagating coupled long waves are out of phase, with the crests/troughs of the internal wave located beneath the troughs/crests of the surface wave; (b) The westward propagating coupled long waves are in phase, with overlying wave crests/troughs.
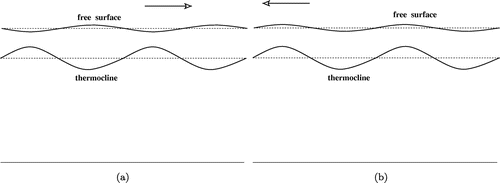
Table 1. Main wave characteristics: The proposed theory predicts the speed and the amplitude ratio of the coupled waves at the surface and at the interface, while field data provide estimates for typical amplitudes of the oscillations of the thermocline and of the surface wave.
Table 2. Field data indicating the main characteristics of the underlying currents.
Figure A1. Typical vertical profile of the equatorial current field in the Pacific region (in bold) and a piecewise linear approximation (dotted broken line). The horizontal axis points from West to East, along the Equator.
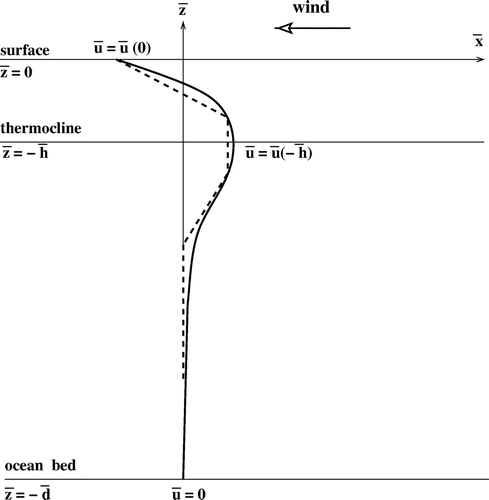
Figure C1. In the moving frame, the critical points of the stream function are surrounded by closed streamlines (Kelvin’s “cat’s eye” flow pattern). The critical layer, depicted by the dashed curve, is the hallmark of flow reversal, the flow being uni-directional away from the critical layer.
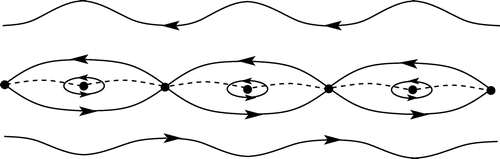
Figure E1. A typical background temperature distribution in the equatorial Pacific, depicting the range of temperature (in C) as a function of depth: temperature gradients are very small below 1 km, while beneath the thermocline, which corresponds to the 20
C isotherm, lies a region of large gradients and above it the depth-dependence of the temperature presents a linear trend (see Gill Citation1982, Weisberg and Hayes Citation1995).
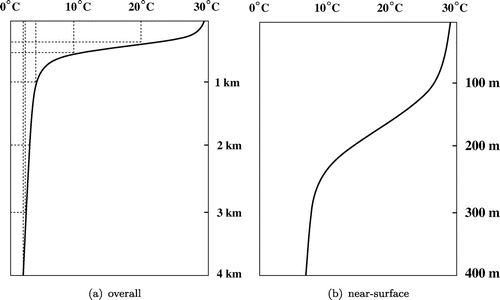