Figures & data
Figure 1. Metabolic Scheme of benzbromarone in rat and human liver microsomal systems (Kitagawara et al., Citation2015). Metabolites M4, M5 and M6 were previously reported (McDonald & Rettie, Citation2007; Kobayashi et al., Citation2012).
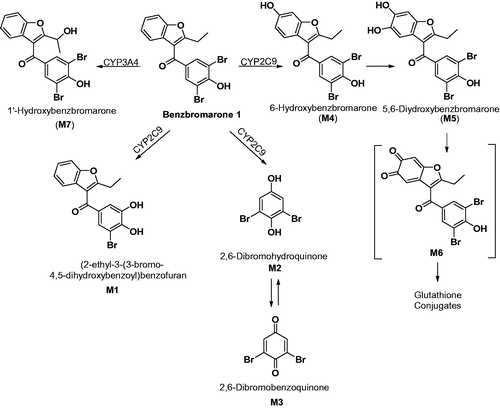
Figure 4. Chemical structures of AZD9819 (1) and its rearranged product 2 (Gu et al., Citation2015).
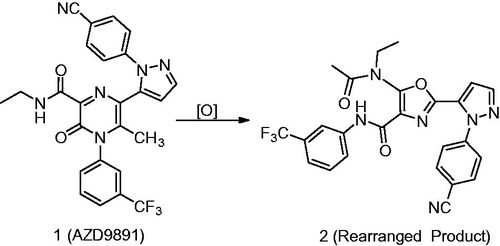
Figure 5. Step 1: Mechanism of oxidation of 1 to the epoxide (3). Step 2: Rearrangement of epoxide (3) to the corresponding oxazole 2 (Gu et al., Citation2015).
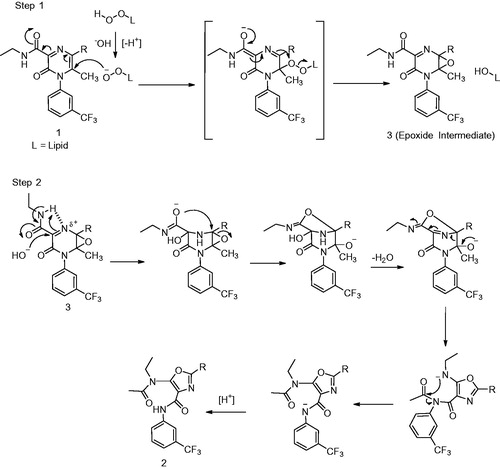
Figure 6. Proposed metabolic scheme of 1 in humans (Meng et al., Citation2015) and chemical structure of linezolid (2).
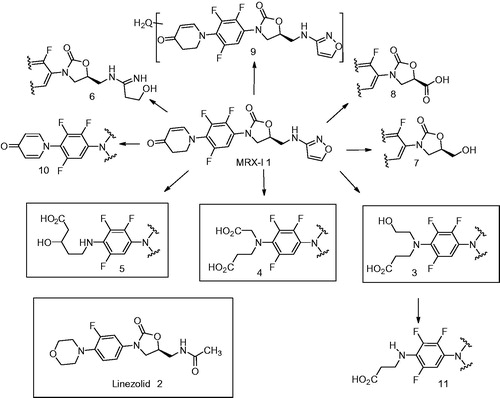
Figure 7. Mechanism of formation of metabolites 3 and 4 by FMO-catalyzed metabolism of 1 (Meng et al., Citation2015).
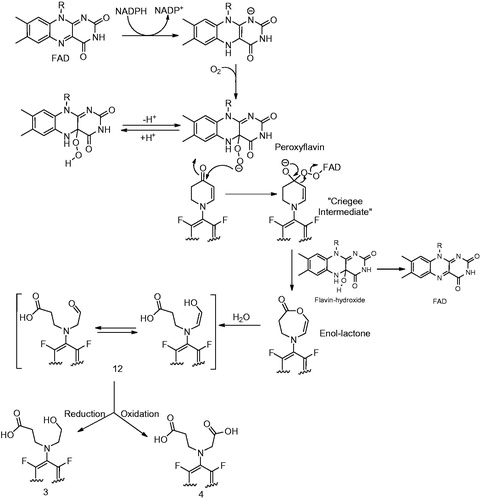
Figure 8. (A) Formation of metabolite 5 (Meng et al., Citation2015). (B) Alternative mechanism for the formation of 5 from intermediate 15.
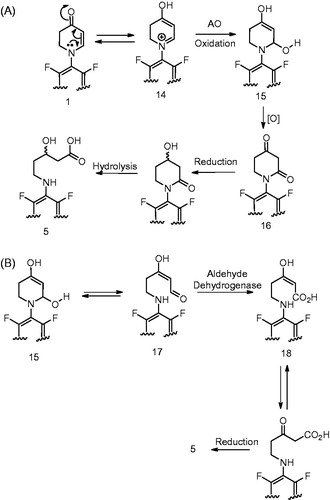
Figure 12. Regio- and stereoslective glucuronidation of NNAL and (R)-NNAL-O-Gluc/(S)-NNAL-O-Gluc ratio in incubations with human liver microsomes stratified by UGT2B17 genotype. *1 refers to the wild-type UGT2B17 allele; *2 refers to the UGT2B17 gene deletion allele. ‡, p = 0.012.
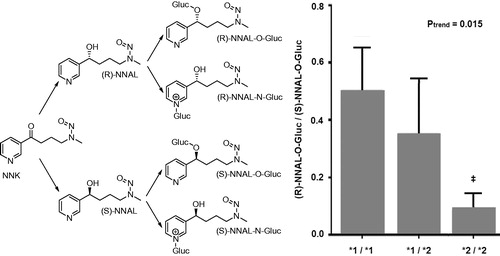
Figure 13. Proposed mechanism for •OH production by tetrachloro-1,4-benzoquinone (TCBQ) and organic hydroperoxides (ROOH), forming a trichlorohydroperoxyl-1,4-benzoquinone (TrCBQ-OOH) intermediate, which can decompose homolytically to produce the •OH and the trichloro-hydroxy-1,4-benzoquinone radical (TrCBQ-O•).
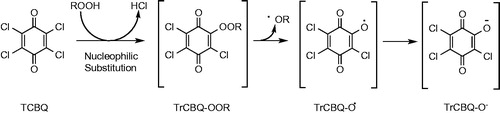
Figure 14. Hepatic metabolism of β-asarone. Formation of the epoxide is suggested to represent the bioactivation pathway to a (geno)toxic metabolite.
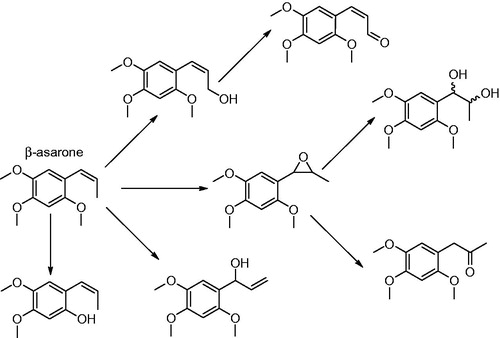
Figure 15. (A) Catalytic conversion of delamanid to M1 by albumin, (B) proposed mechanism of this reaction proceeding via a nucleophile (Nu) from albumin, and (C) the chemical structure of pretomanid.
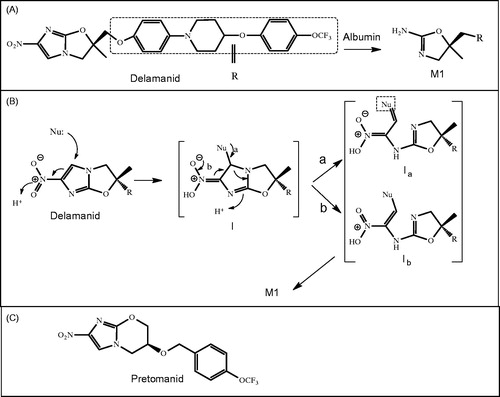
Figure 17. The structure of MEK inhibitors that include hydroxamate side chains, similarly to GDC-0623.
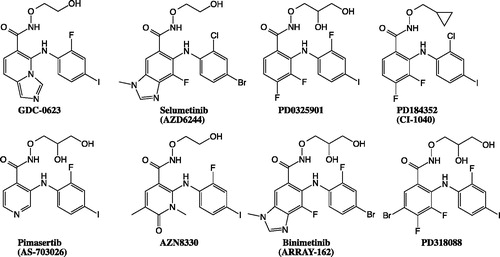