Figures & data
Table 1. Articles covered in this review.
Figure 1. Bioactivation pathway of empagliflozin to 4-OH-CTA. Adapted from Taub et al. (Citation2015).

Figure 2. (A) Structure of givosiran and its metabolites. (B) Structures of (A) unmodified, 2′-O-methyl (2′-OMe), 2′-deoxy-2′-fluoro (2′-F), and phosphorothioate (PS) modified nucleotides; (C) triantennary GalNAc linker from givosiran.
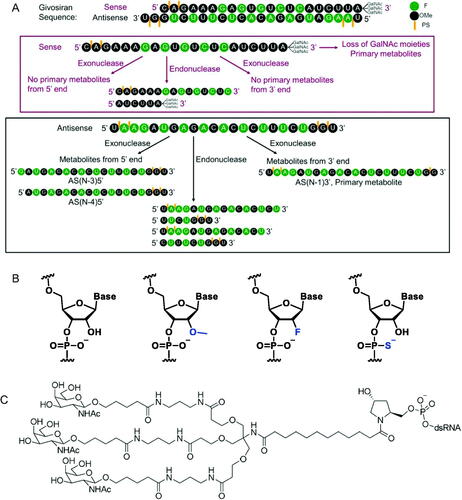
Figure 3. Known biotransformation products of ligand-conjugated siRNA and proposed biotransformation mechanisms: B, RNA base adenine, guanine, cytosine, or uracil; R, 2′-ribose modification, i.e. 2’-F or 2’-OMe. SS is sense strand and AS is antisense strand. Figure from Humphreys et al. (Citation2020) (Copyright 2020, American Chemical Society).
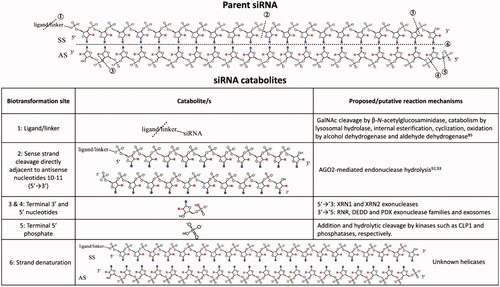
Figure 4. Carbazeran enzymatic conversation to 4-oxo-carbazeran aldehyde oxidase (AO) was chosen as a selective probe substrate for the contribution of various tissue in its metabolism.
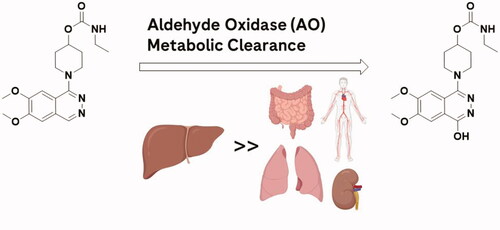
Figure 5. JAK inhibitor that displays poor lung exposure due to AO metabolism (left) and a JAK inhibitor lacking AO susceptibility that displays high lung exposure (right). Arrow indicates site of AO oxidation.
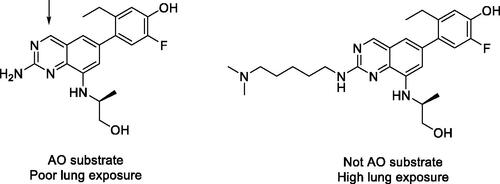
Figure 6. Evaluating the ability of in vitro CYP3A time-dependent inhibition (TDI) data to predict clinically significant drug-drug interactions (DDI). AUC: Area under the plasma concentration-time curve; kinact: maximal inactivation rate; KI: time-dependent inhibition constant; Ki: reversible inhibition constant.
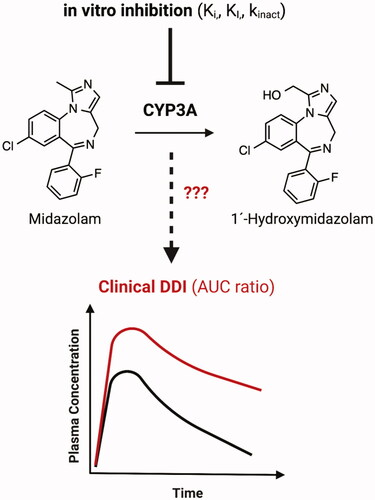
Table 2. Relevant equations used by Tseng et al. (Citation2021) for DDI predictions in static Model 4.
Figure 9. Known metabolic pathways for the oxidative cleavage of nonsteroidal anti-inflammatory prodrug nabumetone to its biologically active metabolite 6-MNA. ALDH: Aldehyde dehydrogenase.
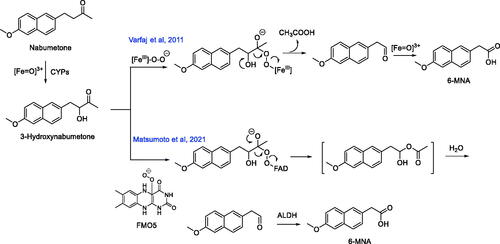
Figure 10. (A) Identification of the potent and selective NaV1.7 inhibitor DS-1971a via optimization of the previously disclosed benzenesulfonamide chemotypes from 1 and PF-05089771. (B) Major metabolites of DS-1971a in animals and human.
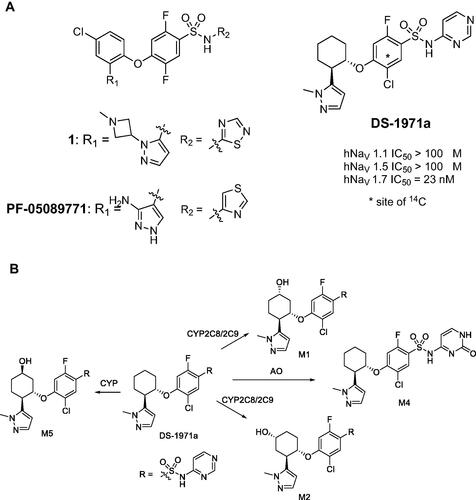