Figures & data
Figure 1. Location of the study area and highlights of the two-dimensional cross-section, including the maximum extent of the Champlain Sea (data from Comeau et al. Citation2013).
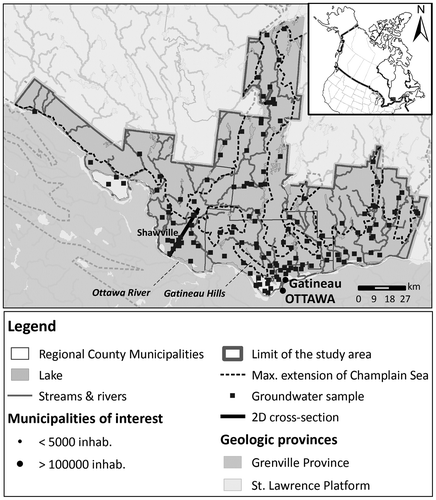
Figure 2. Conceptual hydrogeological model along the selected two-dimensional cross-section perpendicular to the Ottawa River at far right (Montcoudiol et al. Citation2015).
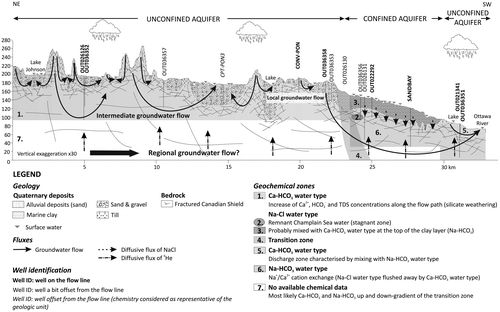
Figure 3. Sequential modelling strategy: (a) Step 1: steady-state flow model, (b) Step 2: calibration of the flow model using tritium, (c) Step 3: simulation of chloride transport from the Champlain Sea invasion, and (d) Step 4: groundwater age model. For simplicity, the inclined bottom boundary is not shown here. Fixed heads, representing surface water, are shown as small triangles on the water table.
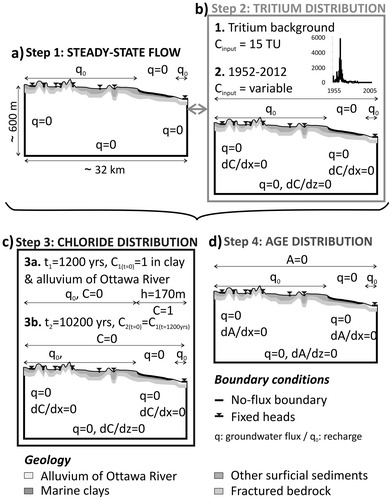
Table 1. Description of the hydrostratigraphic units and their hydraulic properties.
Figure 4. Simulation domain showing: (a) distribution of hydraulic conductivities along the two-dimensional cross-section, and (b) selected mesh detail in three areas near the fluvio-glacial sediment-clay interface. The vertical bars represent the wells shown in Figure 2. Asterisks identify wells where hydraulic conductivities (shown in bold) were calculated from hydraulic testing. For clarity, the full depth of 550 to 600 m is not shown. K distribution is shown for Step 3b (following seawater retreat; K for Step 3a – during seawater intrusion – was identical but had low-K marine clay instead of the high-K alluvium near the far right boundary).

Table 2. Literature review of measured hydraulic conductivities and porosities of Champlain Sea clay deposits.
Table 3. Comparison between observed and simulated tritium concentrations.
Figure 6. Simulated flow system showing: (a) steady-state flow lines, and (b) detail of flow systems in the unconfined aquifer, including total residence times from recharge to discharge, shown on corresponding streamlines (streamlines are identified by streamfunction contours in m2.s−1, e.g. 1E-06; residence times are shown in years, in bold text, e.g. 3 years). For clarity, the full depth of 550 to 600 m is not shown.

Figure 7. Simulated chloride distribution from t = 0, representing the start of the Champlain Sea invasion, showing (a) Step 3a: Simulated Cl after 1200 years of seawater invasion (about 10,200 years ago); (b) intermediate step at t = 2000 years (800 years after seawater retreat); and (c) Step 3b: current conditions 11,400 years after the start of sea invasion (10,200 years since seawater retreat). Selected streamlines are also shown (with 10× less groundwater flux in each successively deeper streamtube. Points 2, 4 and 5 are located on the same streamline which passes through the confined aquifer. Vertical exaggeration is 45×. For clarity, only the local area impacted by the seawater is shown. Concentrations are based on a maximum seawater concentration of 15 g.L−1 (Catto et al. Citation1981). Blanked areas represent concentrations < 250 mg.L−1 (drinking water standard in Canada). See Figure for concentration breakthrough curves at selected points 1–6.
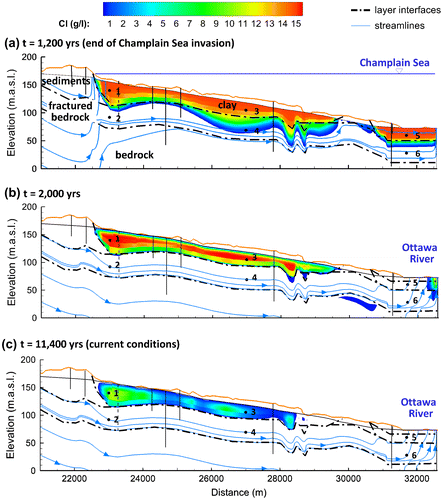
Figure 8. Simulated steady-state mean groundwater ages, showing (a) the two-dimensional cross-section to a depth of −100 m asl, (b) age detail in the upgradient part of the unconfined aquifer, and (c) age detail in the confined aquifer with overburden and rock interfaces shown for reference (dashed lines).
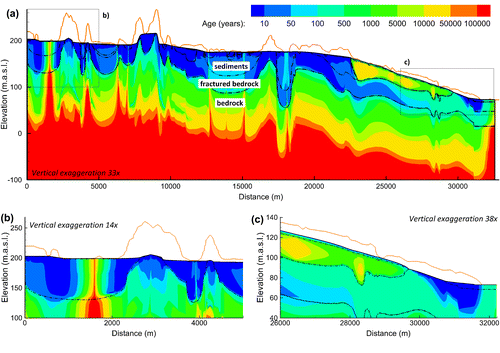
Figure 9. Simulated advective-dispersive groundwater age and tritium profiles for selected wells: (a) OUT036352, (b) OUT036357, (c) CONV-PON, (d) OUT036358, (e) OUT036353, (f) OUT036356, (g) SANDBAY, (h) OUT036351 and (i) OUT021341. The simulated ages and tritium concentrations represent average nodal values based on the open borehole length for bedrock wells, and the entire well length for wells in Quaternary sediments for which the location of the screen is unknown. 14C ages are expressed as percentage of modern carbon (pmC).
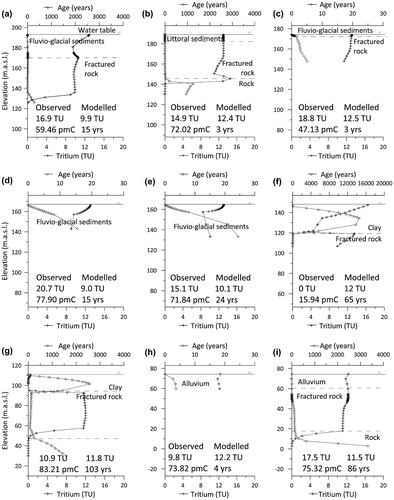
Figure 10. Final revised conceptual model, showing flow directions and total residence times from recharge to discharge zones along selected conceptual flow lines.
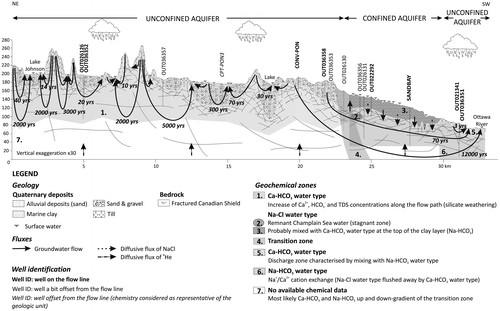
Figure A1. Simulated hydraulic head profiles within the two-dimensional cross-section in different hydrogeological settings: (a) discharge zone under Lake Johnson, (b) recharge zone, (c) artesian conditions, (d) non-artesian conditions in the confined aquifer, and (e) discharge zone under the Ottawa River.
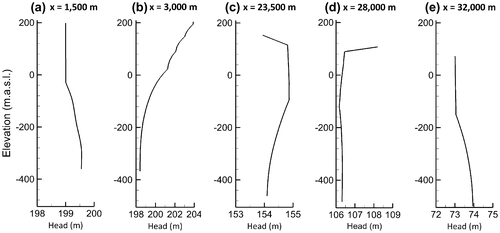
Figure A2. Simulated chloride variations over time at different points in the model showing response and decay since flooding (t = 0) and retreat (t = 1200 years) of the Champlain Sea: (a) chloride response within the overburden (points 1 and 3 in the marine clay, point 5 in the alluvium), (b) response within the bedrock (points 2, 4 and 5 are located along the same flow line), and (c) chloride variations over depth at 1200 and 11,400 years at x = 27,000 m (passing through points 2 and 5). See Figure for point locations.
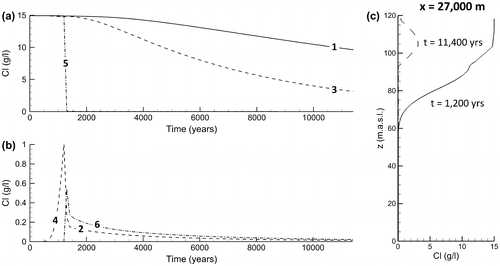
Figure A3. Simulated age profiles along the two-dimensional cross-section in different hydrogeological settings: (a) in a discharge zone under Lake Johnson, (b) in a recharge zone, (c) in the confined aquifer, and (d) in the discharge zone below the Ottawa River.
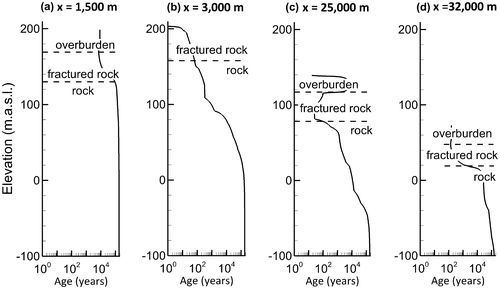