Figures & data
Figure 1. A. Illustration of the classical “Disease Triangle,” incorporating the plant, pathogen, and environment as factors influencing diseases. Recent research on the phytobiome suggests a fourth dimension, encompassing microbes involved in symbiotic relationships. In such a perspective, pathogens and symbionts form complex communities termed pathobiome and symbiome. The plant holobiont (plant and associated microbes) acts on three compartments: phyllosphere (above-ground surface), rhizosphere (region influenced by root secretions), and plant endosphere (internal tissues colonized by the endophytic community). B. Carbohydrate composition of cell walls in two different phyla of plant pathogenic fungi (Ascomycota and Basidiomycota) and Oomycetes. The composition values are presented as weight (%) of the dry cell wall [Citation8, Citation19–27].
![Figure 1. A. Illustration of the classical “Disease Triangle,” incorporating the plant, pathogen, and environment as factors influencing diseases. Recent research on the phytobiome suggests a fourth dimension, encompassing microbes involved in symbiotic relationships. In such a perspective, pathogens and symbionts form complex communities termed pathobiome and symbiome. The plant holobiont (plant and associated microbes) acts on three compartments: phyllosphere (above-ground surface), rhizosphere (region influenced by root secretions), and plant endosphere (internal tissues colonized by the endophytic community). B. Carbohydrate composition of cell walls in two different phyla of plant pathogenic fungi (Ascomycota and Basidiomycota) and Oomycetes. The composition values are presented as weight (%) of the dry cell wall [Citation8, Citation19–27].](/cms/asset/bb48955d-1bd3-444b-a542-4a402a751534/ibty_a_2370341_f0001_c.jpg)
Table 1. Main cell wall components of filamentous plant pathogens.
Figure 2. A. Plant Cell Wall (CW) dynamics associated with the zig-zag model of plant immunity. Glycan-related Pathogen-Associated Molecular Patterns (PAMPs), including complex carbohydrates in fungal and oomycete CWs (chitin, β-glucans) and their cell wall degrading enzymes (CWDEs), initiate PAMP-Triggered Immunity (PTI). Additionally, the hydrolytic activity of CWDEs releases endogenous molecules recognized as damage-associated molecular patterns (DAMPs), triggering DAMP-Triggered Immunity (DTI). PTI and/or DTI induce plant CW remodeling and pathogenesis-related (PR) proteins expression (e.g., plant glycosyl hydrolases targeting pathogen structures). However, pathogen-secreted proteins can limit and suppress PTI/DTI responses. In turn, plants can counteract with effector-triggered immunity (ETI) response, inducing lignification and programmed cell death. B Shows CW thickening and callose deposition localization in the plant cell upon PTI/DTI triggering. C Details papilla localization between the plasma membrane and plant CW to block pathogen penetration. Letters represent processes related to degradation (D) and remodeling (R), associated with the plant (green) or the pathogen (red).
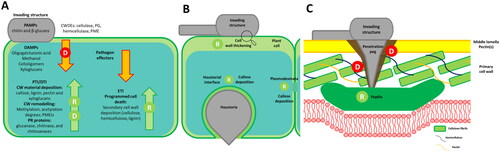
Figure 3. A. Localized degradation of the plant cell wall, involving cell wall degrading enzymes (CWDEs) like pectinases (e.g., pectin lyases, pectinesterases), hemicellulases, and cellulases, is crucial for haustorium establishment in biotrophic organisms. Moreover, -omics studies have revealed the role of pathogen CW remodeling mediated by enzymes (1,3-β-glucan transferases, chitin deacetylases and chitin LPMOs) implicated in plant immunity suppression. B. To penetrate the plant cell wall, necrotrophic fungi secrete a diverse array of CWDEs, including glycosyl hydrolases catalyzing glycosidic linkage hydrolysis (e.g., polygalacturonase, xylanases, α-D-glucuronidases, α-L-arabinofuranosidases, α-D-galactosidases, β-xylosidases, and β-mannanases). Esterases (e.g., pectin methylesterases and acetylesterases) often assist these enzymes by removing esterifications, usually from pectins and hemicelluloses. Letters denote processes related to cell wall biosynthesis (B), degradation (D), and remodeling (R), associated with the pathogen (red).
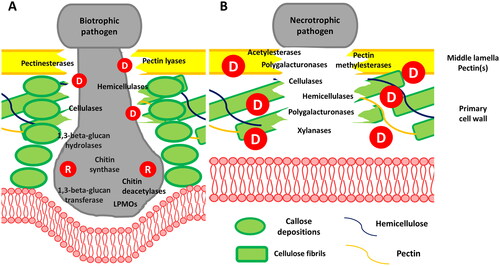
Table 2. Synthetic microbial communities (SynComs) and consortia employed with success in mitigating diseases arising from F. oxysporum, M. oryzae and P. infestans.
Table 3. Potential microbial traits of interest for plant-protection phytobiome engineering.
Figure 4. A. “Smart” symbiome role in the pathogenesis process. The proposed model of the plant disease pyramid highlights the crucial role of the "smart" symbiome in the pathogenesis process. It considers glycome biosynthesis (B), degradation (D), and remodeling (R) in each organism cluster (pyramid corner), directly influencing plant glycome and health. Positive traits induced in symbiotic organisms can counteract pathogen traits, benefiting plant health and enhancing immunity. The "smart" symbiome operates on two levels: inducing resistance (e.g., mediated by endophytic fungi) and activating functional traits (e.g., Cell Wall Degrading Enzymes – CWDE) in response to pathogen-related compounds. Circle sizes indicate health representation, with larger circles indicating overrepresentation and increased health, while smaller circles signify underrepresentation and reduced health in each organism group. B. Simplified representation of bacterial circuit(s) able to detect plant or pathogen-derived signals to readily tune transcription (promoter) and translation (ribosome binding site) level to control expression of target genes. C. Overview on future research “hotspots” in glycopathobiome and phytobiome engineering for enhanced biocontrol.
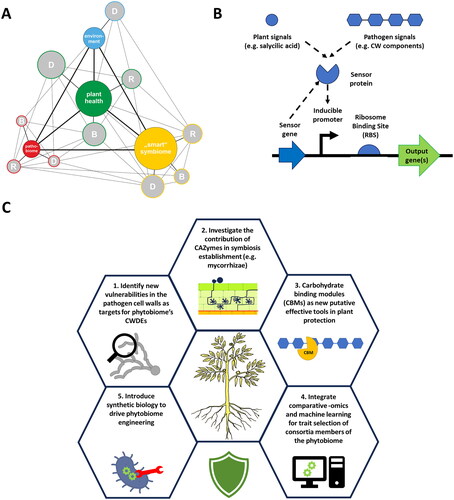