Figures & data
Table 1 The location (latitude (°N) and longitude (°W)), lake surface areas (SA), maximum depths (Zmax), total phosphorus concentrations from water samples collected at spring or fall overturn (TP), and the mean end-of-summer volume-weighted hypolimnetic dissolved oxygen concentrations (VWHO) for the study lakes used in the development of the paleoecological and empirical models. Dissolved oxygen measurements are based on 1–4 annual profiles collected for each of the study lakes between 15 August and 15 September. VWHO was calculated for each year from profile data and bathymetric maps provided by the Ontario Ministries of Natural Resources and the Environment. TP concentrations were obtained from water samples collected at spring overturn in 1990–1992 or at fall overturn in 1996 or 1998.
Figure 1 Regression models of (a) chironomid-inferred mean end-of-summer volume-weighted hypolimnetic oxygen concentrations (VWHO) and (b) VWHO predicted using the empirical modelling approach versus VWHO calculated from measured profile data averaged over at least 3 years. The dotted lines represent 1:1. r2 jack and RMSEPjack are the jackknifed coefficient of determination and the jackknifed root mean square error of prediction, respectively. SE is the standard error.
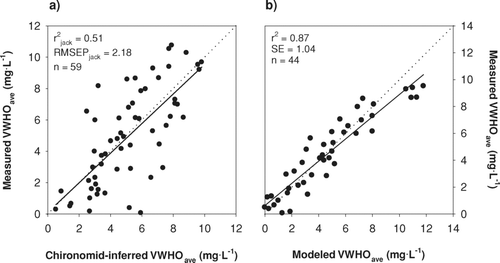
Figure 2 A comparison of mean end-of-summer volume-weighted hypolimnetic oxygen concentrations (VWHO) predicted using the paleoecological and empirical modelling approaches. The models are compared in their prediction of present-day (left panel) and background (right panel) VWHO. The dotted lines represent 1:1.
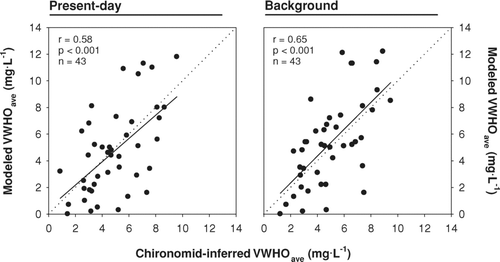
Figure 3 A comparison of the change in mean volume-weighted hypolimnetic oxygen concentrations from background levels, predicted using the paleoecological and empirical modelling approaches.
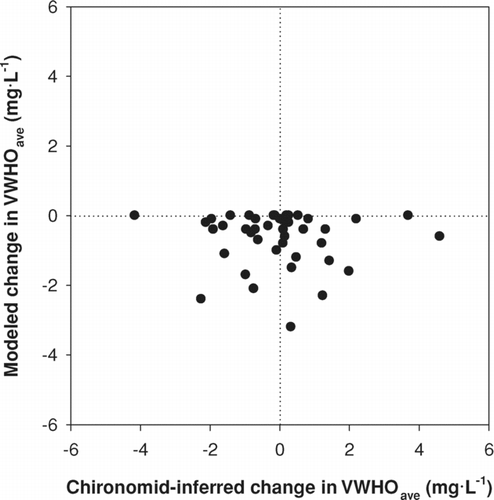
Figure 4 Conceptual diagrams showing the theoretical changes in mean end-of-summer volume-weighted hypolimnetic oxygen concentrations (VWHO) from background to current (present-day) levels. In Scenario 1 (left panel), a net increase in VWHO is caused by a multiple stressor effect resulting in an increase in VWHO (e.g., a rise in water levels that increases the volume of the hypolimnion) minus a decrease in VWHO associated with increased shoreline residential development (i.e., single stressor effect). In Scenario 2 (right panel), a net decline in VWHO is caused by a multiple stressor effect resulting in a decrease in VWHO (e.g., climate change resulting in increased strength and duration of stratification) and an increase in shoreline residential development (i.e., single stressor effect).
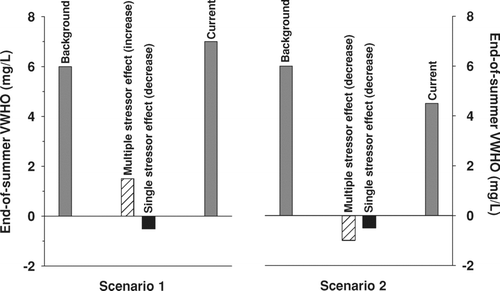
Figure 5 Long-term monitoring data (1976–2005) from Chub Lake in central Ontario showing a long-term decline in total phosphorus concentrations, concurrent with a long-term increase in mean end-of-summer volume-weighted hypolimnetic oxygen concentrations (bottom panel). These changes have occurred despite an increase in the number of shoreline residential developments from 1978 to 1997 (top right).
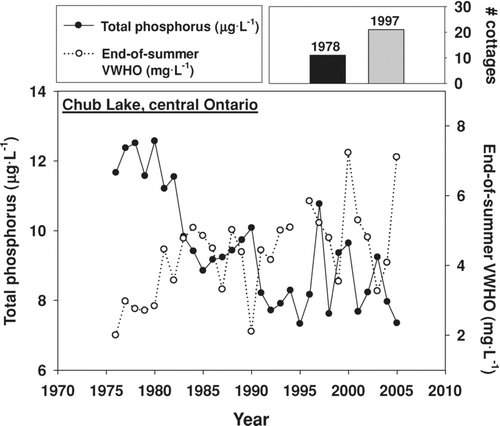