Figures & data
Figure 1 A plan view of the surface indicating the adatom and interstitial sites used in this paper. Ti atoms are dark grey and O atoms light grey, with larger atoms closer to the surface. The bridging oxygen rows are indicated with the dashed lines. Site A (and equivalents A′ and D) is the adatom in its most favourable site, next to two bridging oxygens and one in-plane oxygen. Site B (and its equivalent E) is the adatom in its second favourable binding site next to one bridging and two in-plane oxygens. Site Fi is an interstitial site one O–Ti–O layer down; there is an alternative interstitial site Bi, underneath B. Interstitials another O–Ti–O layer down are labelled Fii and Bii, respectively.
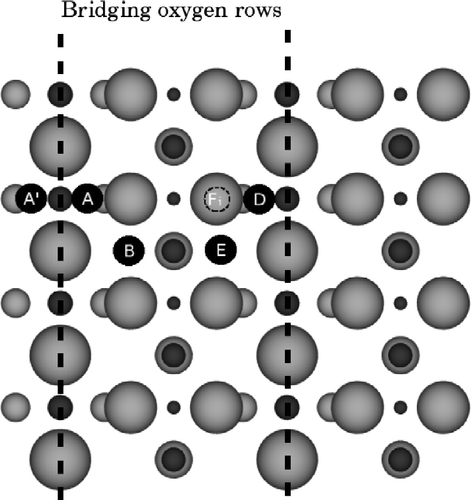
Figure 2 Charge distributions induced by the layer 1 interstitial site Fi: (a) DFT+U calculations using Bader analysis; (b) the original Hallil QEq potential and (c) the fixed oxygen-charge variant of the QEq potential. O is light grey and Ti dark grey. The radius of the ions reflects the excess charge ( − e) the ions have compared with the bulk environment (referred to the ions at the bottom of the picture). To set the scale, the interstitial in (b) has an excess of 0.42e− , and its six nearest neighbour oxygens each have an excess of ∼0.3e− , over bulk values. Only a section of the full cell used in the calculations is shown for clarity, with the upper surface being the free one with the exposed bridging oxygen.
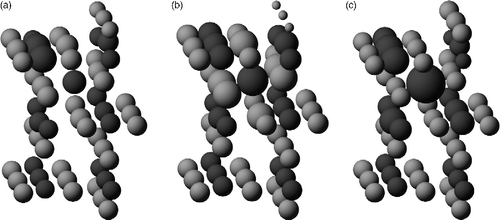
Figure 3 The energy landscape of the adatom and interstitials in the B/Bn (o) and A/Fn sites (*),where layer 0 corresponds to the adatoms and layer n to the interstitials below n O–Ti–O surface layers. The energies are given relative to the most stable adatom site for the model, which is site A in all cases.
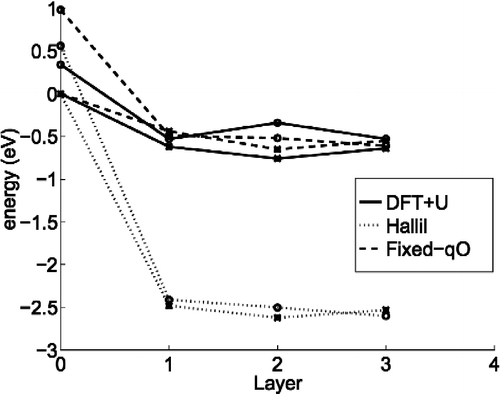
Figure 4 NEB pathways for (a) A–Fi via exchange with a surface sixfold coordinated Ti; (b) B–Bi via exchange with a fivefold coordinated surface Ti and (c) B–Bi direct move without exchange. Here, the reaction coordinate is the Euclidian distance along the path from the initial state.
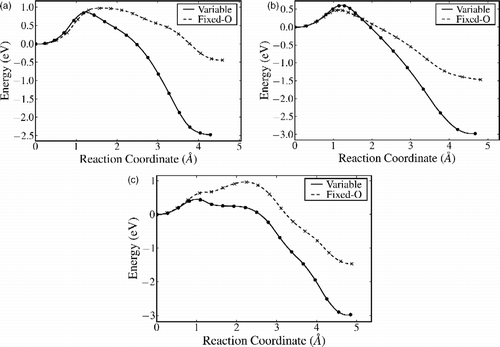