Figures & data
Figure 1. Universal laser-heating head (UniHead) Equation(1) with a π -shaper Equation(2)
mounted for single-crystal X-ray diffraction experiments in DAC at the ID09a beamline at ESRF (Grenoble). Equation(3)
The optical fiber connected to the 100 W laser light source, (4) the silver-coated carbon mirror, (5) the DAC (green arrow in inset shows the direction of the laser beam; color online).
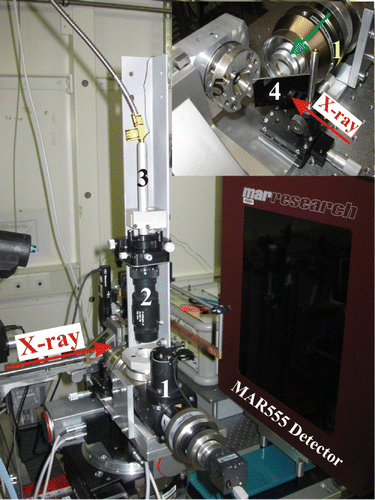
Figure 2. Splitting of the Ne (111) line during heating at 48 GPa. Pressure was calculated according to Citation23.
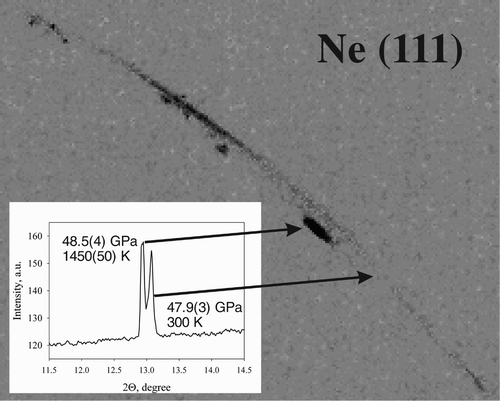
Figure 3. Unit cell volume of tungsten measured at different pressures and temperatures. The green data points were used to fit a thermal equation of state with Ne as a pressure gauge Citation23, as described by EquationEquations (1)–(3) Citation15. The pressures of the red data points and for the curves were determined using the fitted thermal EOS parameters. The inset shows the difference between the values of the measured unit cell volumes and the values obtained from the thermal equations of state in the present study (blue plus symbols) and the values predicted based on ab initio calculations (pink crosses) Citation22 (color online).
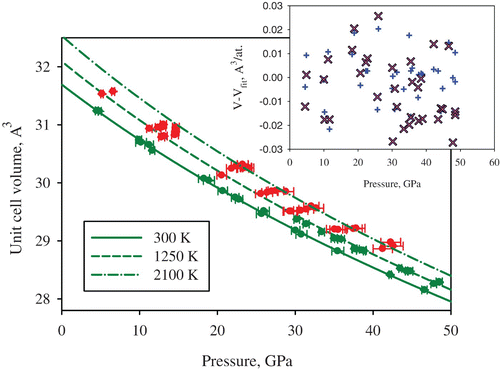
Table 1. Thermoelastic parameters of tungsten (Equations 1–3).
Table 2. Results of single crystal structural refinement of Fe2O3 at different pressures and temperatures.
Table 3. Results of single-crystal structural refinement of (Mg0.62, Fe0.38)(Al0.36Si0.64)O3 silicate perovskite (space group Pbnm) at different pressures and temperatures (A=(Mg 0.62Fe0.38), B=(Si 0.64Al0.36)).
Figure 4. Pressure–volume equations of state of (Mg0.62, Fe0.38)(Al0.36Si0.64)O3 silicate perovskite as obtained by means of single-crystal X-ray diffraction experiments in a laser-heated DAC. Uncertainties in the molar volume and in pressure (determined from the lattice parameters of Ne Citation23) are within the symbols. Continuous curves are the fits of the experimental data using a third-order Birch–Murnaghan equation of state for ambient temperature (blue) and for 2050(100) K (red). The inset shows the variation with pressure and temperature (filled symbols, ambient temperature; open, 2050(100) K) of the normalized pseudo-cubic axes of orthorhombic perovskite (a*=a/√2 (V/4)−1/3, b*=b/√2 (V/4)−1/3 and c*=c/2 (V/4)−1/3; a, b, c and V are the unit cell parameters and the cell volume, respectively) (color online).
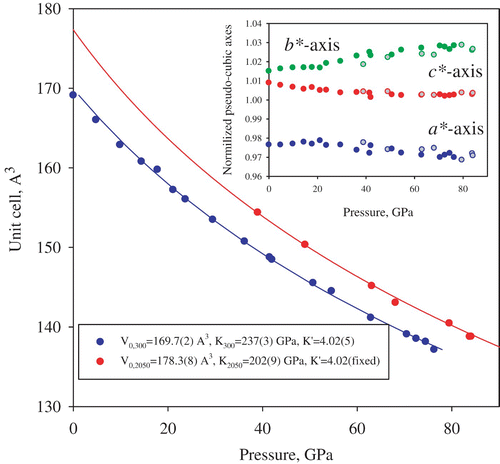
Figure 5. Pressure and temperature dependence of the mean bond distances of (Si, Al)O6 octahedra (a) and of (Mg,Fe)O8 polyhedra (b) in (Mg0.62, Fe0.38)(Al0.36Si0.64)O3 silicate perovskite.
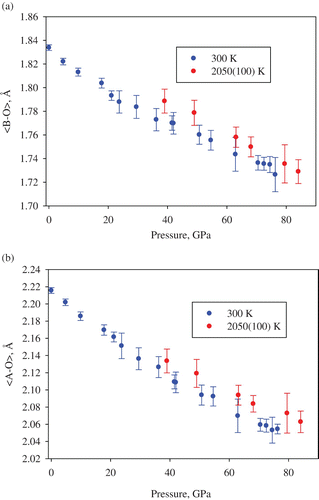
Figure 6. The variation of B–O1–B and B–O2–B (B\dbond Si, Al) angles in (Mg0.62, Fe0.38)(Al0.36Si0.64)O3 silicate perovskite with pressure and ambient temperature (a) and at 2050(100) K (b).
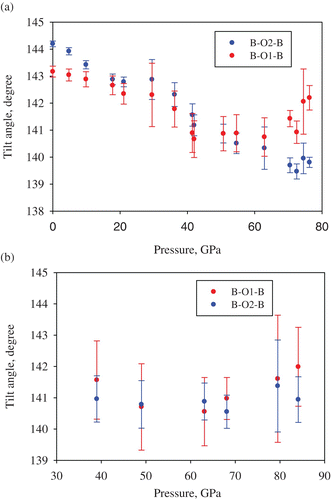