Figures & data
Figure 1. Penetration depth at a temperature of 268 K shown as a function of frequency for dry snow with 0.5 mm grain size, wet snow with dry snow density of 0.4 g/ccm, freshwater ice, and impure ice. Dry snow penetration depth is calculated with two different models, where the main difference is that the Tsang model accounts for grain size.
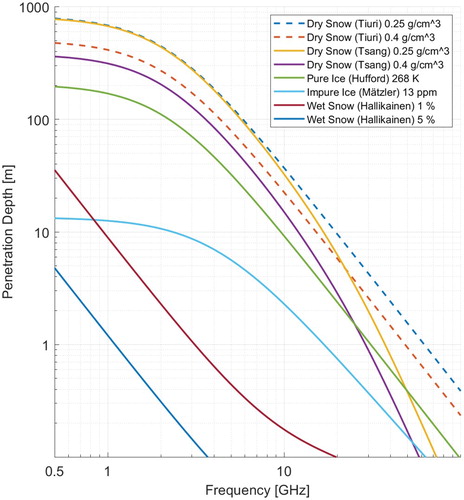
Table 1. UWiBaSS key characteristics.
Figure 3. Close up of Vivaldi antenna (a), radar system with dual Vivaldi antennas (b), and the same radar system mounted under a UAV (c). (a) Close-up of modified Vivaldi antenna, with resistive loads (1), inserted slits (2) and printed lens in aperture (3). (b) Dual Vivaldi antennas mounted in bistatic configuration (painted black) and (c) Antenna prototype (and radar box) mounted under “Cryocopter FOX”. The antenna angle regulation mechanism have a slight angle when not powered up, to keep tension on stabilizing bungees when pointing in nadir.
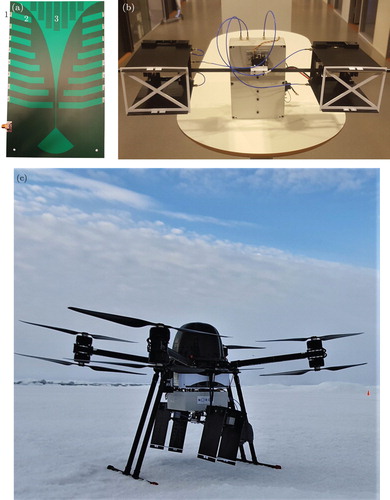
Table 2. Comparison of single Vivaldi antenna and dual modified Vivaldi antenna.
Figure 7. Example showing radar image and UAV altitude with the UAV mostly flying outside the unambiguous range, and entering the ambiguous range at approximately 150 s.
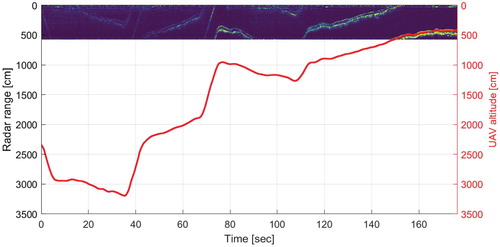
Figure 8. Returned power compared to the theoretical returned power according to the radar equation. Data was collected with a drone-mounted radar with a max unambiguous range of 5.7 m.
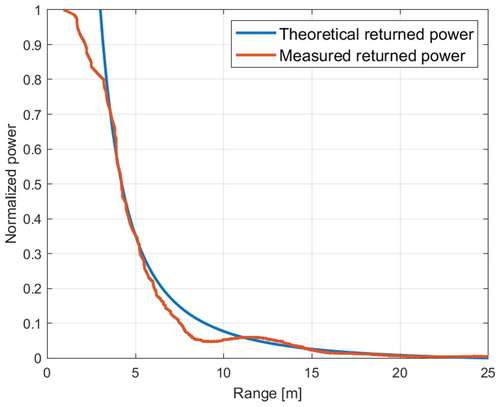
Figure 9. The same data as in Figure , before any correction (top) after altitude dependent power calibration (middle) and finally after the shifting procedure (bottom).
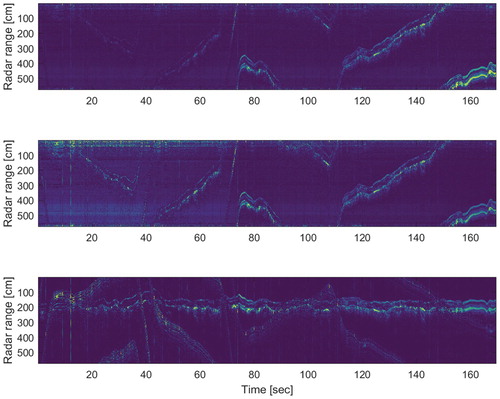
Table 3. Ambiguity windows.
Figure 10. Pixel variance of the un-calibrated (top) and range calibrated (bottom) radar image. Ambiguity windows are marked with red diamonds.
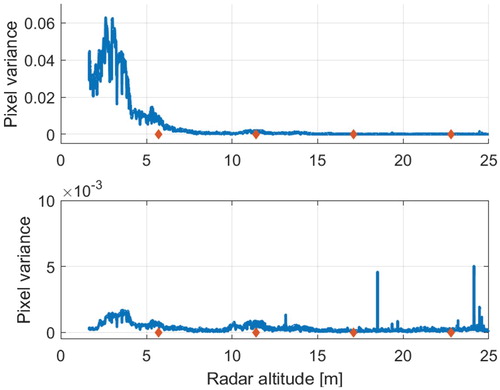
Figure 11. Chart of preferred zones of altitude given different target lengths. This is calculated for a radar system with 5.75 m unambigous range.
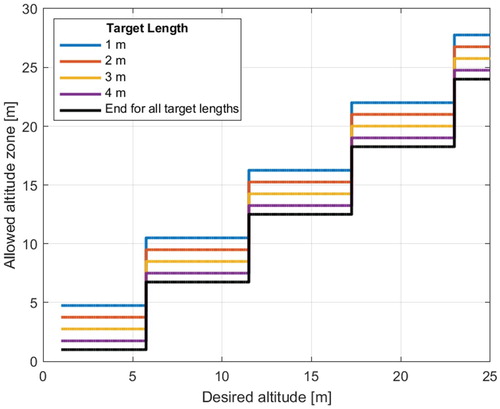
Figure 13. B-scan radar image from site “High res. 1”, with interpreted radar snow depth compared with in situ snow depth. The radar measurement is a 100 m transect with 38 manual measurements over nearly 80 m. All data points are geo-referenced.
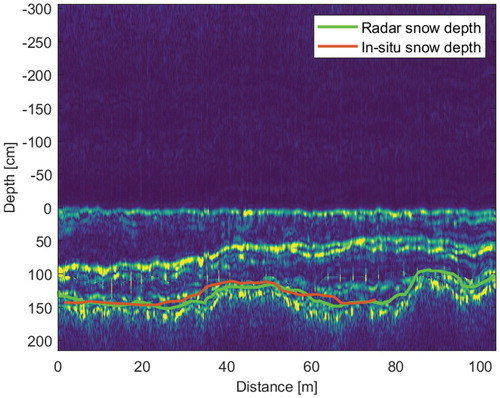
Figure 14. A-scan radar responses in red (150 slow time averages), compared to in situ stratigraphy in blue, assessed using the “hand test” [Citation43] shown with the top x-axis. (a) Site 4 and (b) Site 8.
![Figure 14. A-scan radar responses in red (150 slow time averages), compared to in situ stratigraphy in blue, assessed using the “hand test” [Citation43] shown with the top x-axis. (a) Site 4 and (b) Site 8.](/cms/asset/860aaba7-c77d-44d4-b426-99d9d24ff81e/tewa_a_1799871_f0014_oc.jpg)
Figure 15. Georeferenced snow depth from site 2, measured with drone mounted radar (a) and GPS snow probe (b). and root-mean-square error (RMSE)
cm. (a) Radar snow depth and (b) In situ snow depth.
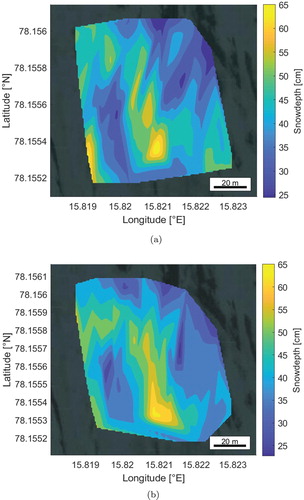